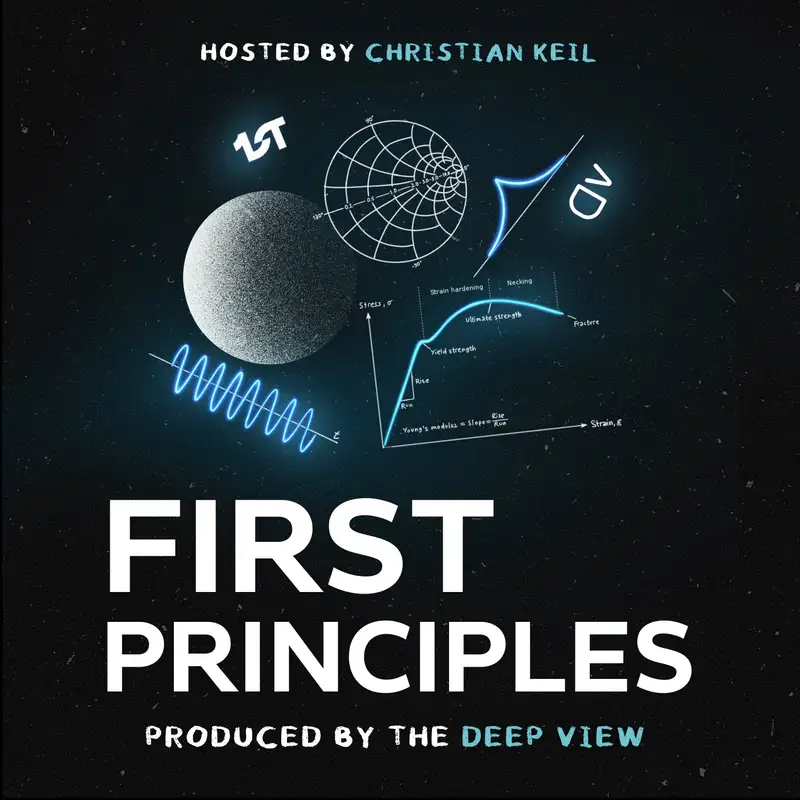
#14: Quantum Computing Isn’t Dead Yet | César Rodríguez, Commutator Studios
Maybe it would help to talk about Twitter, which is actually how we found each other.
So I think it's fun that the coolest connections are made on Twitter.
I happen to just reach out and say, hey, I need to do a quantum episode. I
want to talk about quantum computing. And then out of all the recommendations, you
popped up as one of the people that was the perfect people to talk to. So why don't
you tell us who you are and why you might have risen to the top of the quantum
Yeah, yeah, yeah. So I'm Cesar Rodriguez. I'm the CEO and founder of
Computator Studios. I've been working on quantum computing for over 20 years.
This is kind of my life's passion work. To
be honest, the reason why I was one of the last ones on Twitter is because Twitter
used to be a really thriving quantum community, and it kind of shattered the
What happened? I'm a lot changed in Twitter. I don't have
to go through the drama that's happening. Everybody knows. For example, the
I refuse to use that. It was really a lot of communities kind of died out. And that
was one of them. And I'm kind of sad about it because I miss it. It was great
Oh, geez. So did people go elsewhere? Did people go to like these like
There's no good place. I feel very lonely. A lot. It
used to be very thriving. We would have huge debates about papers and
there was drama and gossip. It was super
Why don't you set the scene for us? Just tell us, what is quantum computing and how
This is kind of like the school of thought that I belong to. School of
thought is filled with history, right? So before we used to think the
universe is made of particles and fields. Now we think it's made of information. And
so you can go back to the Matrix movie or something. If you have enough information,
you can model the universe kind of thing. Well, it's kind of wrong, the matrix, because it
was using bits, and that's not enough to model the
universe. And that's fundamentally what quantum computing is, is
that the universe is fundamentally quantum mechanical, and
classical information, that's the one that you can represent with bits, it's just
a tiny part of the whole information contents of the universe. So
what powers quantum computers is just the rest. A visual image
I want everybody to keep in mind is like, bits, if you think of like the
planet Earth or something like that, So if the planet Earth,
a bit will be like zeros and ones. So you could think of it like the South Pole and
the North Pole, for example. And so if
you try to describe everything in the universe, just
using those points, it's very limited. However, quantum computer allows
you to use the whole sphere, even inside. So it's much, much
more information content than that. But of course, it contains the
old stuff. That's like super, super important. And the idea is
that with that, that allows you to do computational
things that were essentially impossible. So
with quantum computers, you can solve problems that we thought were practically impossible
to do with the biggest supercomputer in the world. That's ultimately what it is.
These are some very important problems, like my favorite one
is chemistry, because chemistry is fundamentally quantum mechanical.
Atoms are quantum mechanical, of course. That's how physics started. Quantum
physics started. It was a debate. What's more fundamental, chemistry or
physics? And when quantum mechanics came in, physics won,
which I'm very proud of that. Because then suddenly the
periodic table, you can derive it from the quantum mechanical principles.
But from that, the fundamentals of quantum physics is
that you can use this description of the universe, which
is, of course, very odd. It's very counterintuitive in many ways, to
do more than you could do before. So, for example, if
you want to understand how a molecule works,
let's say for pharma or for medicine or for new materials, you
have to understand the quantum mechanics. And computational, that's
really, really hard. It's super difficult. However, the
way it works usually is that you take the quantum mechanical problem, you
approximately heavily, like literally crop it up, go with
a machete to cut it off, and then you
put it in the biggest supercomputers in the world, which a lot of them are used for this kind
of problem, and you evolve it in time with also very approximated methods,
and then you run out of money, so you stop the run. That's
basically it, because it's like limitations, right, from compute power and so on. And
then when you're there, you kind of try to approximate back up to the quantum mechanical
level. And of course, that's extremely inefficient and
incomplete. This is why when you think of, let's say, a
chemist, you think of somebody with a lab coat mixing
like cocktails, right? You don't think of somebody like me that programs
quantum computers. And those people are basically people
with PhDs doing very, very sophisticated trial and
error by mixing cocktails, right? It's extremely inefficient
to do discovery that way, and it's because they couldn't do it from first
principles. And quantum computers will allow us to do a lot of that, so really narrow
down all the possibilities for this, because it's just better understanding
And so is there a piece of the quantum computer that, like, the reason it
works is because it is the same substrate or whatever
as the thing that it's trying to figure out? Like, the universe is quantum, If
we make computers quantum, then they can just directly simulate things in
the universe? Is that basically the idea? Or is it a compute thing, though? So, like, there
are two versions of it that I could see. Like, version one is it's the substrate. It's the
same thing. That's why it works. Version two is quantum
computers are just plain more powerful. Like, you can do 2 to the n instead of, you know,
like whatever classical computing would have in terms of bits. So,
if we did develop a sufficiently big classical computer, are
there things that still a quantum computer could only do, like an arbitrarily
large classical computer? Could it do the same sort of things as quantum, or
is there something fundamental in the quantum nature of how things are
From my philosophical point of view, they're both exactly the same, because I
believe the universe is made out of information. And so they're the same.
It's like, what is the description of the universe you're going to have is what we can compute, ultimately. That's
really, I think, of course, this happens to be from my generation of scientists, right?
Like, this might change for my son when he's a little older, right? But for now,
that's not how we see. So for me, they're exactly the same. And ultimately, think
about it, if you want to model the whole universe, which is a pretty darn
big place, you will need a quantum computer bigger than
the universe to do it. And so it has to be a quantum computer. If
you want a classical computer to do it, it will have to be exponentially
larger, most likely. So ultimately, it's both. It's
really the nature of reality such that it's quantum mechanical. And
in classical mechanics, it's just extremely incomplete to
do that. And an analogy I really like to use is
trains versus airplanes. And so classical computers are
like trains. You know, like, they're very fast, they go on rails, they're really
nice. And quantum computers are like airplanes. They
allow them to fly, so there's more dimensions you can play with. Of course, it comes
with a lot of challenges. Piloting an airplane is much more harder than
being a conductor of a train. But you can go to new dimensions, and
you can go to new places, and you can see things from another level. So that's
something I think is very fundamental. It's like, so
what's the best description for planet Earth? I mean, Trace gave
you a pretty nice one, but there's of course much higher level descriptions that we
need. No matter how big the supercomputer you build, it
will not be able to solve certain basic problems for quantum computing. This
The other piece of context that I would like to give, just in the very beginning,
is the fundamental challenges of why quantum computing is
even hard in the first place. I do think that people sort of
know this too. Like they've heard of maybe like coherence. Maybe they've heard of like the
fact that these super computers have to run super cold or like these quantum
computers have to run super cold. Do you mind talking about that? There's like the very high
Yeah. So it's really that the same physics that made them so powerful
made them very frail to errors. You
can't separate those two things. It's just like airplanes, the same physics that allows it
to fly makes it prone to storms and turbulence. There's just no... You
just have to accept that it comes with it. So a lot
of people are like, but why do you put rails in the sky? I'm like, you're missing the point of
the airplane, right? The point of the airplane goes to places you could go with rails.
It's like really, really crucial for the airplane. It's not just the speed of the airplane. It's
like it doesn't need the rails. That's so, so dramatically different. So it's
the same with physics. And the main physical principle that makes
it so, so so difficult to translate these things,
it's called the no-cloning theorem. This was done by Wooters
and Swerk. They are kind of mentors of mine. I really look up
to them. When I was at UT Austin, I
was in the office. They used to be grad students, so I remember all of that. And
the no-cloning theorem, it sounds really odd, but when
you think of bits, you know, zeros and ones, the main thing we do is you
can look at them and you can copy them. You can say, oh, I
just read one, so you write it on a piece of paper, one. They call it
cloning. You're just copying the information. However, with quantum computer, when
you look at the qubit, you destroy the information. You
can get a one or zero, but you don't get the full qubit superposition thing.
And you can still do a lot before you get to that point, but
that's really, really fundamental. You cannot copy all of it. So that
makes it fundamentally very difficult to manage errors, because how
do we manage errors? If you have a hard disk, you
have a RAID system, what do you do? You just copy many times the same bits. You
just keep copying them. They'll be fine, right? But you have them both to do
that, which is not possible in quantum computing. But that
same principle that prevents us from copying the qubits is what
powers the computational advantage of quantum
computers. You can't have one and not the other. They
come together. So why are they so
frail? And this is, of course, like, why does the
universe look classical as opposed to quantum mechanical? Why
don't we see superposition with our own eyes? And ultimately,
of course, I started saying this, the universe is quantum mechanical, but
it doesn't seem like it. It does seem like the matrix. It does seem like it's made
of bits. So what's going on there? It's a big gap between them. So
this distinction is called quantum decoherence. Like, how
do you go from the quantum mechanical perspective onto the
microscopic perspective that we use, which is the one that it kind of
looks like bits for the most part, except when you have it to what I'll dig deep
into things. And quantum decoherence happens, it's
an emergent phenomena, because since
quantum information cannot be copied, It's not very
robust. While classical information can. So when I look at
something, I get a little bit of classical information and then in my brain it's being copied and
I make it so I can have a complicated image, I put it on the computer, I put
it on the internet, it's like I make that it goes into your brain, right? And
so it's really that. It's really that. The classical part is easy to
copy, so that's why we see it all the time. It's more robust. It's a
little bit like a Darwinian thing. that allows
it to survive all this noise. And the classical is
very, very fragile. But this one, the
way I like to think about it is that why do we see the
world as classical? Why is it so counterintuitive for people?
And I think really it's kind of our own fault. It's
not that quantum is wrong. It's that our brains evolve to
manage saber-toothed cats. Like, you see a saber-toothed cat, your brain
knows how to run, right? It's like, it sends all the information, you just
run. It's not designed for understanding Schrödinger's
cat. And that's really why it's so difficult for
us to understand, is that you need additional, a
level of abstraction that our brains are used to. Because it didn't evolve for
that, but it doesn't mean that the universe is not
Hey everybody, quick break in the action to tell you about Coda, who
is today's sponsor for this episode. Coda is the best way to keep your team
all on the same page, literally. It consolidates spreadsheets and
documents and anything else that you need to do planning for a remote,
distributed team. This is perfect for a team like First Principles, where
we have people that are scattered across the country. Not everybody's based here
in San Francisco that works on the show, and so we have to stay collaborative in some way,
and that way is Coda. The simplest example is just choosing a set of
tasks that you have to distribute across a team. We can see what progress
everybody's making, whether people are on or off track, and we can just keep them all
in one place. We don't have to have them scattered in a bunch of different programs. If you're
a product manager, you can do your OKRs through CodeUp. If you're a leader
at a company, you can just make sure that all of your disparate teams come together in
one place. And you don't have to start from scratch. Coda has this huge document
library that you can leverage to just get started and not have to start from
a blank page. If you want a platform that helps your team work better together, head
to coda.io slash fp today for a free
trial. Let's get back to the show. isn't there a piece of it too that's just
like the law of large numbers too like the fact that like the universe
is quantum like the things in this water bottle technically are you know
quantum like all the little individual atoms are both a bit quantum mechanical not
classical rules but there's so many like billions,
trillions, quadrillions of atoms that are in this thing that I
can pretty much know that my finger's not going to go straight through when I tap the top
of it. I mean, there's like a statistical element to the
scale at which humans operate that we don't even have to worry about the quantum
stuff. It just like takes care of itself because it's all averaged out. Isn't that also
Yes, that's a big part of it, that's a big part of it. Of course, there's a way, like, when
you show me the bottle, I can see the colors, and that is a quantum mechanical phenomenon.
The colors are clearly quantum mechanical because it has to do with the resonance of
each individual atom, and the reason why I see some parts being white, some parts
being red, some parts being black, is really because we really
see the quantumness of this model. But, of course, when you push
the finger, you're like, no, I can't describe the Maxwell equation, it just repels each other.
So there's some truth to that. In terms of the law of large
numbers, I want to talk about thermodynamics, because
that's kind of where you're getting into. So of course, thermodynamics says that when you
have stuff that's really big enough, there's like
a limit on infinity that you go to some sort
of average of something. But there's a process for
this. It doesn't happen immediately, although in the thermal class, we like
to pretend it does. It's not true. And it takes
some time. And the rate of this happens is
different from classical information and quantum information. So quantum
information, it's usually way
faster to decay than the classical information, basically. And
so that's really why the universe looks so classical. It's
that the quantum state, the classical information does get averaged out, right? Like
if you leave a hard disk in the sun for hundreds of years, the information is
going to be deleted. Well, the qubits do the same, which happens much
faster. But it's basically the same. It's really the same. It just
happens at very different rates. And this ratio of how fast
the quantum information dies versus the classical information, this
is really what we call the coherence. It's not just Information being lost
is the ratio of the classical versus the quantum. That's really, really what we
Interesting. But what's the fundamental reason why that has to
be true? Why can't we just build quantum
bits that are very robust? And why do we have to have our quantum computers be
so, so, so cold to not get randomly thermally messed
Yeah, yeah. So I'll answer it two ways. First, the historical one,
and then the little more modern one. So the historical one, it's called the measurement problem.
And so maybe some of your audience might have heard about it, which is
you have a quantum mechanical thing, and then you look at it, you measure it. That's
how it's called. That's how Heisenberg and Bohr
and so on came up with it. You measure it, and suddenly you destroy the
information. It happens instantaneously, and suddenly it looks perfectly
classical. That's really, and the measurement at that
time, it was like a really magical thing that connected the
quantum world from Isenberg and Schrodinger's equation to
the classical world of particles and waves
that we know. Like it's really, it was really thought of
something magical, and they would say, of course, shut up and calculate, don't ask those questions.
Of course, that was over 100 years ago. So we have learned a little bit
since then and we don't think that way anymore. And
the way we see it is like really the world is fully quantum mechanical. So
the measurement process itself, the measurement apparatus, they
always used to tell you, yeah, well, the measurement apparatus is like you. You're really microscopic. And
you look at one atom with your eyes. So because they're big and they're
small, you destroy a lot of information. We do think that. We
do think that in some way. But it's not instantaneous and not magical. It's
a dynamical process of coupling quantum mechanically with quantum mechanical equations.
And it just happens to be that as we do so, because
of the no-cloning theory, which is something super fundamental about
the universe, that you cannot copy certain kinds of information, but others you can.
So no-cloning is for quantum information, but the classical ones you can. So
if you want to think of the planet Earth picture, it's
like you could have copied the whole planet Earth sphere, but
if you tell me the North Pole and South Pole, I can copy those too.
If you're in the North Pole and South Pole, I can tell you, I can copy that right now, but I don't have
the information for the other latitudes. That's kind of how it works in quantum mechanics. So
ultimately what we think is that you cannot copy some
kinds of information. So the ones that are very robust to these measurements, which
means robust means it doesn't get erased, which means you copy,
is the classical thing. So the way to think about it is that we're very quantum
mechanical. The atom is very quantum mechanical. We inspect
it, but for it to get the information to get into our brain, it has
to be amplified. And the only stuff that can be amplified is stuff
That makes sense. I think the thing that always struck me as really weird
about the whole double slit experiment thing was the voodoo of
observation and the atom or the particle, the
photon knows that we're watching it or whatever. But what you're saying
is that the photon isn't looking back
at us and knowing when it gets observed. That's not actually the thing that is causing the
weird interference pattern. It's something else. It's the more fundamental nature
So the more modern way we understand the measurable problem is that it's
an emergent phenomenon. that the classical world that
we see, the pits, it's really emergent. It just happens to
be very, very robust emergence. I suppose, and the other stuff gets
kind of washed out, it just becomes fluctuation. So that's really, really, it's like,
we just, we just are, we're just really good at seeing that stuff, not the rest. So
it's a little bit of a bias from us being humans, but it
does seem like it's a pretty good bias because we are computational machines
and it does make sense we focus on the stuff we can copy because that's what they do.
All the way from Turing, that's what he focused on. What are the things you can copy?
Does this mean then that... So that's a really good background, I
think. Hopefully people at least have a base level understanding of
what is all this stuff now. I think let's hop now into more of the, what are the
modern things that people are thinking about with quantum computers? How are we building them differently now?
What are the real challenges that we're facing with them? And maybe a simple way
to kick this off and sort of bridge from what we've been talking about, Does
this nature of qubits, this reality of what a
qubit is versus what a bit is, does this mean that the solution can't
be a hardware one? No matter how good we make the hardware of
our quantum computers, there's just going to be these errors because there's
Yeah, yeah, yeah. So something I want everybody to think about
is when you look at a news article about quantum computers, they
always mention how many qubits the computer has. And
just by itself, that number is completely meaningless. And
so I want everybody, when they just see the title, just the number of qubits,
I want everybody to be very skeptical of everything written below it. Because
it's usually, it means it's probably very superficial understanding of
what's happening. And so really, of course, there's a lot of parameters that matter.
I like to put them into three categories. I think they represent enough.
Of course, it's still a simplification. But one is the number of qubits. The
other one is like another axis. That one will be the
connectivity between the qubits. And the third one is
the quality of the qubits. So think of it like a three-dimensional axis. Like you
want all those to be very, very good. You want a
lot of qubits. You want it to be very well connected in a
very controlled way. So for example, I am made of qubits and
you are made of qubits. You're made of about 10 to the 20, 20 something
qubits, right? That's a lot, but you are a terrible quantum computer. No,
no, no, no offense. I'm also a terrible one. And
it's because your connectivity is not optimal for that, and your gates
are not the best for that, because your gates are built for something else. I mean, for keeping
you alive, right? Which is a pretty good thing, right? We appreciate life. But
you need to do all three very well to have what's called a scalable quantum
computer. And of course, there's pros
and cons of all those, and you can do some trade-offs between them.
For example, if you have qubit one next to qubit two next
to qubit three, but qubit 1 and 3 are not
directly next to each other, you can sacrifice the one in the middle to
set information between them, for example. But that makes sense, right? A little bit of
a telephony. So in some way, you can swap number of qubits for
connectivity, is what I'm saying. And also, there's ways that
you can exchange number of qubits for the quality of the
qubits. So this is known as quantum error correction, which
is you use many qubits to support one qubit. So
think of it like one of those human pyramids of
cheerleaders or something, that you have many people holding one up, right? Oh,
that's very high. Oh, my God, it's very high. But you need all the people to be very good at holding
them and keeping them stable. So that's another one. And so all
the hardware modalities, what's important to understand is they
are not good at all three. Nobody's good at all three. Nobody
has achieved that. When we achieve that, that's going to be, of course, the golden moment
in quantum. It's going to be scalable. But
everybody's good at some of those, but not the others. And they're trying
to figure out a path of compromises to get to scalability.
And nobody has figured it out. So there's a
lot of modalities for quantum computers. I'm going to focus just like this is
too many for me to list. But just
some categories of the most common ones, the ones that are easier to find online
to program stuff. Some will be atoms and ions. If
atoms and ions people are listening, I know you're very afraid, don't get offended, but
if we just say category, please, I like you very much. But
both of them, the strength is, they can put a lot of qubits, and
the connectivity is quite good, and also the error rates are
really good. However, there is a limitation of
how much you can scale that up. And this is
really the fundamental challenge, it's like, that one might work very well now,
but it's unclear how it'll work in the future. And the reason is, they
are literally putting atoms, or ions of course, ions are just like, you
take an electron out of an atom, And they're putting in
a vacuum chamber, so this is like more vacuum than empty
space. And they're having them surf electromagnetic
fields, so literally lasers or electromagnetic pulses, it's
crazy. They just have them surf each other, and they have them all
hanging out on the waves, like a bunch of surfers on the beach. And
then they make them dance together when they need to do the calculation. That's how they do
it. This is amazing, right? But this is incredible that
we can, we have this level of control of individuals. I don't like, this is
unbelievable, like science. There's been many, many prizes for
this stuff. It's incredible. But this is in
a vacuum chamber. And a vacuum chamber, it looks like, if
you think of kind of like a World War II movie and you think
of a diver, he has this big helmets. with
the glass panes and it's metal they look kinda like that and
they literally in that thing they have to put the lasers and the
electromagnetic fields and the atoms and the vacuum pump and
they have to have them all dance together and ultimately the limit is
how much can you cram into this thing this
is a special limitation of the electronics and the lasers and all that so
you can see that even if you can put a lot in one it's
easy to ask what's the limiting factor because If
you build this chamber bigger, then the alignment
of everything is harder, the backing system is much harder, and this
is a fundamentally hard thing. It's hard to picture how you can keep
scaling this up and up with the current technology. So those
are really good now, but the scaling is harder. On
the other hand, you have these other quantum computers that are called superconducting, they
use superconducting qubits. And so they use squid devices. And
some of those are like IBM or IBM, for example, even D-Wave tries
to do some of those. And those are
different in this three-axis thing. So they're
probably going to be really good at having a lot of qubits, and they're going to scale nicely.
The connectivity is kind of in the middle of the road, like, it's okay, it's
not... And the reason is because they
can't fit more, but it's okay because they can build many,
so they can swap middle qubits if they need to, like, they can sacrifice
a bunch if you need to. But the quality of the grates is
not so great compared to the others. But what's
nice about them is that the way this is built, all the superconducting qubits,
it does rely on a lot of similar technologies to silicon
fabs. It's not the same, but it's all similar. So it's easy to
picture. If you can build 1,000, you can build 1 million. This
is not a crazy thing. And the challenge of that is
that to prevent them from quantum decoherence, instead
of before in atoms and ions, you just isolate them from
all the observations and the rest and thermodynamics by putting them in vacuum. For
those others, what you do is you still put them in vacuum, but mostly what you
do is you cool them to as close
to absolute zero as you possibly can. And
so that's Bayard. I mean, it's hard in the sense that
there's a physical limitation of what can there be,
which is liquid helium. A
lot of the prices are just for like super
cooling. So we know the limits of what this can do. But
it does seem like there's a fundamental limitation of how much you can cool
the superconducting chip with this. And if you
have looked at photos of this kind of quantum computers,
Yeah, yeah, yeah. They're crazy looking. It's the one that Joe Biden was
And he was looking at the wrong part of the computer, by the way. I love that
Yeah, he was looking at the top, which is where the cooling system is, and the sheet
was at the bottom. So it's a little bit like, oh, my God, this computer is
very impressive, but you're looking at the fan of the computer. Yeah. But
in some way, it's because you can tell, the chip is tiny. All
this other stuff is super obvious to tell with your eyes. But the issue is, you
need to take all these pipes and cables that help cooling, and
you have to fit them to this tiny chip, and they don't fit. It's
like a really visually stunning image that this is like, why
are we using this steampunk machine to build a plotter computer?
It's because they don't fit. There's no other way around it. So this is
the fundamental limitation for those. is really the
the cooling systems and there's a the physics we know
the limits we're really good at that but it's an engineering challenge of
how do you put more stuff to cool them in there's not enough space to
do it so but if they figure it out we start getting better and
better then it's easy to foresee that you could scale this chip much much
larger And there's other modalities too, there's
quantum dots, there's photonics, there's a lot of other modalities. And
they also light differently in this qubits connectivity
and quality scale.
Nobody has figured it out. We're all trying everything we
can to figure it out. Some of the things I mentioned are hardware, but I
do think fundamentally that a lot of it's going to be software that's going
Interesting. So are there differences between the atoms and ions
and the superconducting versions in terms of interconnection? Are
either better or worse at the interconnection step of the
Yes, so so the gates the gates in atoms and ions are
really really good quality And the information is quite robust. They're
a little bit slow. That's what they always say. So they always They're
always having a little like fast on the furious argument the people from squid and
and the people from from atoms and ions But ultimately, uh,
ultimately, I don't think this that Speed is the ultimate
limitation. It's the scaling. It's really that the
atoms and ions is you have to put them in back of the chamber It's a little bit hard to picture how
you can connect two vacuum chambers without creating a
lot of noise and a lot of other problems. While the other one, it's just
basically, the model will be more like printing a circuit. So that does
I think this is a good time to talk about gates, basically. Like, what are
they? I mean, maybe it'd be helpful to ground people in like, what is
a gate in terms of like classical computing? And how does that sort of build
classical computing? And then how do they translate into the quantum world?
Okay. Yeah. So, so we can go a little bit by time to ancient
Greece and Aristotle, right? Nice. So,
so, so, uh, classical computers, of course, use logic. Uh,
COs are false, true or false, that this was encoded in Greek times.
And we know this as, as classical logic. And, and, and if
Aristotle came from the past into the, into now, and he saw,
if you explain him how a microprocessor work, he will totally understand
the fundamentals. Oh, yes. It just, it just does the computations for the, for
the truth table. He will get that. This will be very familiar
for him. Quantum computers, because they use
another kind of information that's not just true or false, right? It uses other
things. He will not understand that. This will make absolutely no
sense for him, and he will be very upset about this, probably
draw a sword or something at you. And
so, of course, what is a gate in classical computers? Let's
say you have a one, and you can turn it into a zero, and a zero to a one, that's
a NOT gate. And you can have two, let's say ones and zeros, and then you
come at AND gate, or OR gate, or an XOR, and so
on. And just from those basic principles, you can build a whole microprocessor. That's
ultimately how we see it. Any microprocessor is always built
off those fundamentals. And quantum computers are a little bit different. In
classical computers, a lot of those gates, if you think about
it, some have the same number of inputs and outputs, like
they're not, but most of them don't. Most have two to
one, right? Like this is a choice that we made as humanity. Like,
oh, it's good enough. If it was good enough for us, it's good enough for us now.
But that does have a thermodynamic cost because it means fundamentally you're
erasing information. And that's why computers eat up. Interesting. Yeah. Because
you have more bits at the beginning than the end. Always. And
that's when computers heat up. I can hear my computer fan going off
right now and sometimes your phone gets warm in your pocket, right?
And that's exactly that. It's like you're destroying information
that increases the entropy of the universe. There's nothing we can do about it.
And so in the 70s, Charlie Bennett
started asking a lot of questions. He was a researcher at IBM, and he's now one
of the founders of Quantum Computer. He was really obsessed with, can
you build classical computers that have the same number of inputs and outputs, just
to save energy? And he came up with models for that. It turns
out you can. We don't do it because, ah, whatever. Chips are
cheap. Transistors are cheap. But in principle, you could totally do it. And
then he started thinking like, huh, then what's the next thing? And
he said, well, quantum mechanics is fundamentally like that. And
that's where he got interested in quantum mechanics. He was not a quantum physicist. He
just wanted to make very efficient computers in terms of energy.
And he started asking questions about that. And he went into
the deepness of quantum that way. And now he's, of course, one of the founders of
the field. And the logic gates
we use for quantum computers, some are similar, but some make
no sense. For example, instead of not, you can have the square root
of not. So if you think of like the
planet Earth and like the globe and the North Pole is
one and the South Pole is zero. So if you do a
not, it's like you flip it around completely so that the
south pole is pointing up now and the north pole is pointing down. That would be a
knot. So in quantum computer, and that's all you can do because the
only thing that matters is what's up and down in classical computer. In quantum computer, you
can put it just sideways so that the north pole and south pole add
sideways. And that would be the square root of knot, for example. And there's a lot more
things that you can do like that, which is really ultimately what
deals with this. What you're doing is you are taking
this quantum mechanical information, getting it
entangled, getting it to very complicated super quantum superpositions such
that you massage them into doing a computation for you
simulating some quantum device and at the very end then you measure it and
you get some probabilistic result And then you do this many,
many times. And if you did it well, you get a
really good probabilistic distribution of what the correct answer is. So
you do everything quantum until the very end. And that's when you measure it.
And then you start getting statistics. And if you try to measure in
the middle, you ruin the computation. It's like, no, no, no, no,
no. You're not allowed to open the oven before the cake is done. If
you do it with a souffle, of course, the souffle collapses, right? So it's like
that with quantum computers. If you do the measurement in the middle, the souffle collapses.
And that's a little bit what happens with this quantum decoherence is that
this loss of classical quantum information to the environment, to
temperature, to the air, to all these things, like this
fragility, it works a little bit like
opening the oven of the souffle a little bit and that destroys
So I know that in classical computing, you can basically take just
those super, super basic building blocks and then demonstrably prove
that you can do anything with them. Like if you have the certain set, it's
like complete. And that complete set of very simple operators allows
you to do anything. Is there an equivalent like completeness set
Yes, yes, yes, yes, yes. So it has been proven that as long as you have
some basic gates that fulfill certain criteria, you can do any computation
with that. There's a challenge to them because The ones
you might build might not be the most robust to the noise. So there's
always like a little pushback. Yeah, in principle they
will work, but maybe you want to build ones that are a bit more robust to the noise. Like,
you know, it's the same that in principle you
can have the human pyramid with the cheerleader at the top with any
Yeah, totally, totally. So basically what you mean is there are
multiple discrete sets of these complete instructions that have been found
or something, and then the question is, and maybe there's even theoretically more
So as long as it fulfills the standard criteria, it's okay. And you can,
it's very easy to compare between them. This is not, it's not a big mistake. Like
you can, if you know one and you want the other, you just use a very simple mathematical
Hmm. What are they? Do they map roughly similarly to
Or are they just super weird? A lot done because it has
Yeah, right. But the not does, but the square root
of not, it's a little weird, but it does also, you know, it's
a little weird, but it works. There's others like Hadamard gate, which does have
a classical analog. But a lot of
them, we like to think about them in terms of rotations. That's why I was mentioning
Leplanier for rotating it. But very quickly, our
geometric interpretation, it just stopped working. Because although
I'm using the sphere for discussing a qubit, two qubits
are not really two spheres. It's a completely different mathematical element
on SU4, and it happens to be a coincidence that I can describe the
qubit as a sphere. So you have to think of them as rotations
on higher dimensional complex space that they have no visual interpretation.
But all of them are really switching around, swapping things around
a little bit in that higher dimensional space. And
this is similar to Fourier transfers, for example. Fourier
transfers are a rotation from, let's say, time to frequency
domain. A lot of the quantum gates, that's exactly why they do.
And there's Laplace transformations are another one that's a rotation. And
there's so many intervals. So a lot of quantum are very
So when you're doing a gate that has more than one
qubit entering or whatever, Do those always
entangle? Is that what it means to have two qubits
go into a gate, is that they entangle with each other and then some crazy stuff happens afterwards?
Yeah, so some gates entangle, and
some gates do other things that are non-entangling, like rotations of the
superposition, created superpositions. However, when you put them
all together, And you look at the quantum algorithm, the quantum program,
we describe the quantum program in terms of gates, which is something
that's super confusing when you think about classical compute. Because
in classical compute, we do not program by building circuits.
We don't. The circuits are already printed in a
fab, right? Somewhere in the other side of the world
always. It's already determined, it's fixed. In quantum, we're always
dynamically reprogramming the architecture. So this is very, very different. It's
something I want to emphasize. But all the
quantum programs, they always have this pattern. You
put the information as bits. You want to encode a program as
something you want to solve. Let's say the energy is a molecule, you put it as
bits. and then you use some gates that entangle all
those bits then you do additional computations with
more complicated gates that kind of shuffle things around and the rotations
and then at the very end you do quantum measurements to get bits out
so you have bits in bits out But of course, when
you have the bits out, this one, you have destroyed the
computation, but you got some information out. And then you repeat this over and
over to build a statistic. So always you start classical
information, then you entangle it. So in some way, there's a layer of
gates to do that. Then you have the middle part that is the actual computation itself.
And then the end is, let's inspect the result. Let's open Schrodinger's cat's
Cool. So this is a good time to transfer into like talking about what these things actually
do. So like what, how are we actually using them today? What are the actual algorithms we
figured out? Didn't know how to run. I mean, it seems like given how
crazily unintuitive it is, like it seems really hard
to even discover these algorithms in the first place. I, I know of like, I
think two, like I definitely know the Shor's algorithm is one of them that, that
can run on quantum. And then there's like, there's like a, um, like
Groves or something is another one of them. Yes, Grover. Grover. Okay,
nice. So like, I know that those two exist. Are there like tons more or
So there's many, there's many, there's many. Of course, you have to think
about this. This is like discovering algorithms like
Alan Turing was doing before he got computers to program, right? in
some way and right now with our computers we never think oh i'm going to
write an algorithm you just program the entire computer and if it works it
works right this is that when we talk about algorithms a really abstracted version
that we have proven prove it to certain limits of of
computability how powerful they are i always like to say that
Complexity theorists are the most unreasonable people you'll ever meet.
And I like them for that. That's why I love them, because when they say
something, they're really, really confident that it will always work, no
matter if it's an absurd situation, it's going to work. So when you talk about algorithms, the
ones that are proven to that extent. So I mentioned one that
I think is the easiest to understand, which is quantum chemistry. Because it's an algorithm, it's
like you take a quantum problem, you map the energies to exactly
the gates to simulate coupling between different energy levels, and
you get out the result of the quantum molecule. And this is called quantum simulation, this
kind of method. And that way, we think that since
the universe is quantum mechanical, we think it should be very, very
efficient. There's no proof for that. But we have good reason to believe that this
is an exponentially hard problem. Classically, maybe this is going to be linear. Maybe it's
going to be very efficient. There's good reasons to think about it, but this
time it's been shown by complexity theorists. On the other hand, you have the
two algorithms you mentioned, which is what is Grubber search. So
search algorithm is the one where you have an unstructured database.
So this is like you have the phone book, but instead of looking by last names
and finding number, you're looking by numbers trying to find the last name. So
you're doing it wrong. So if you
want to be very sure, you have to look at the whole, every page, every number in the phone book,
right? Like all the end. So if you have end numbers, you have to look at all of
that to be sure. And with Grover's, you can do square root of
n, which is crazy. Because that means you don't have to
look at 100, you just have to look at 10, right? And really
the way you do it is that you take all the phone numbers and you entangle them, and then you
do some quantum messaging that increases the probability of the
correct one, so you can read it more often. So that's
Grover's search. That's a really important one. And a
lot of quantum algorithms, all quantum computing has that
one as the baseline. We have very good reason to think
quantum computers will always give you a quadratic performance
improvement over any classical thing. And it has to do with
the nature of the physics of how the wave function works. It's always squared.
There's always tricks you can do. But we want to do a
lot more than quadratic. Quadratic is awesome, but we want to do more. And
that was when Shor's algorithm came about. Peter
Shor was a famous mathematician for IT. I
thought many years later, he's a fantastic guy. He's
always mentioned as, he really destroyed the complexity
theory classes, which I love. I love what people
have said, complexity theorists. And he really tried it because we
really thought that factoring was a very difficult computational
problem. Factoring numbers, so you
have a big number, what are the factors that you multiply and
comprise it? And of course, that's the basis for RSN encryption, which is
the workhorse of all kinds of privacy we use now, like the internet and
so on. It's really the workhorse. Like, you couldn't have working
credit cards. And if you can break RSA, you can steal all the money in the world,
right? Like, this is like James Bond villain kind of
level, right? And like, this is, uh, Fast and Furious 10, kind of like, threat,
Yes, that's right. So 11, we had to go up to 11. I haven't seen 10, I haven't
seen 10, that's why. So the reason is because we thought all
encryption, as we know it now, classical encryption I like to call it, is
based on you make assumptions that your enemy will not have a
computer too powerful. That's your assumption. We
assume you think of your enemy the person you don't want them to look at your text
messages. They couldn't have a computer so powerful to crack this code. And
that was a good assumption for a long time. But what Peter Shaw
did was he shattered that. Because he showed with
a quantum computer, you could very efficiently crack
that. So suddenly what was unimaginable for
your worst enemy now is totally doable. So of course that
has a lot of implications for defense and there's
a lot of geopolitics around it. The story tells itself, right?
And so a lot of people say like, yeah, but it doesn't matter. You can always come up with a new
algorithm and you can have a bigger encryption key. But
that's kind of missing the point. Because the data you had created
10 years ago, they can crack it. They have it because they copied it and they can crack
it. So if you had nuclear codes, they have them now. But
also, it's that the point of
Peter Scholl's algorithm is not that he happened to crack one encryption scheme.
is that assuming your enemy doesn't have access to
powerful computers it's not a very safe thing that's
really really really the message like you shouldn't assume that that's
just stupid like that's just wishful thinking like don't don't don't
put your your deepest national secrets assumptions of
that like it makes no sense it's just too it's just too weak And
of course, for that, there is what we call quantum encryption, which is another
method that uses the same physics I was telling you about, that if you measure
quantum information, you destroy it. So you
encode it in a certain way that if there's an attack, when
they attack it, they just ruin the information. It's gone. They can't ever read it again. And
so that one, what's nice about that one is that, of course, what challenges
that you need qubits, you need a new internet to transfer those qubits. But
what's nice is that the assumption there is that your enemy cannot
break the laws of reality as we know them. That's what's happening that
it's something that I have a very powerful computer, right? So fundamentally, that's
what we think, like, that was very transformative for all of them because
it completely changed their perspective about what encryption should be, what security should be.
That's super, super dramatic. So those are the
big ones. However, there have been a lot of other applications of some
of these algorithms and variations on this algorithm, some changes on
that, and they have a lot of applications for logistics, for
pharmaceutical, for chemistry, chemistry I mentioned, for materials, for
finance, and a lot of them are a little bit based
on the Grover's quadratic improvement. Because
for them, quadratic improvement is actually a lot. That's really significant for
some solutions. So we do think that for some
of these large optimization problems, as broadly
defined as possible, that the quadratic
performance of quantum computers might be very, very useful.
With that said, I expect us to discover way more algorithms in
the future. A lot of the
developments in algorithmic computers was really come from programmers, just trial and error and
figuring out how things work in practice. And so we're at the beginning of
that stage, because we are now working quantum computers. We're still trying to
figure out how to use them in practice, as opposed to
just on a piece of paper to come up with the algorithm. How
many quantum computers are there in the world? I
Yeah, yeah. Less than a thousand, definitely, though.
Less than a thousand, less than a thousand. So
it's a bit hard to say because there are some that are a
little old, so they take them offline, but they still exist. So
maybe if you know the right person and they open the closet door, they
could give you access, but it's a little bit like that.
That is so interesting. I mean, just imagine a world where there are only a hundred computers. Like,
that's the stage rat. That's literally the stage rat. It's like, oh, why is there
not more applications of these things? And like, why is there not more, you know,
algorithms or whatever, more use cases that are really in the world? It's like, well, dude,
there's less than like 100 computers right now. We're figuring things out
And if you talk to anybody in the program quantum computers, the
first thing they're going to always complain is how the queues. You're always waiting
in the queue. You never know when your program is going to run. It's
very inefficiently done. And
the hardware providers, they don't care at this point to optimize the queue. Whatever.
I remember reading back in like 2020 or 2019 or
something that Google had like achieved quantum supremacy. And
that that meant that like, in my mind, what that meant was, oh,
now we have big enough qubit systems that are so big
that they can do calculations that no classical computer could ever do. And
in my mind, what that meant was, okay, now we're quantum supreme or
whatever. We're all just going to have quantum computers now. Like, why would we ever have classical computers?
Because we have achieved supremacy. But of course that hasn't happened
yet. Right. Even though we have these massive qubit systems, like what
even is quantum supremacy? And why did, why did we, why did, uh, like people think
Yeah. So, so I want everybody to think of like, what does supremacy it's
a, it's a very technical term. And what it means is there
is one problem. It doesn't matter which one. that
the quantum computers outperform any known classical computer.
That's what it means. It doesn't matter which problem it is. So it could be
testing your nose by pulling your arm around your head. Something ridiculous
like that. It doesn't matter. So the first step
to show that quantum computers are more powerful is to build quantum
computers that are really good at one toy problem that you made up, hoping
that the classical computers are bad at it. So in some way it's like you're defining
the problem to make it look good, right? You're defining the criteria to
make it look good. And I'm not mocking this, like I'm very good friends with the people in
in Google that achieved that and so on. This is important.
This is an important first step. You have to start there, right? You just
want to come up with a criteria to look better. And so that announcement
from Google was like that. But unfortunately, what happened was that
once they announced the problem they were at, somebody
And there have been other announcements like this from Chinese groups, from IBM, and
this is always happening, this is back and forth. Unfortunately
from the news, they make it seem like every
step is dramatic and a revolution as opposed to continuous grind,
which is what it is. So they're like, oh no,
they think everything is wrong. No, that's how it's supposed to
be. You're kind of pushing it and then classical computers push back.
That's how it's supposed to be. We should expect though that
soon, let's say a year or two, that there's a
clear winner. That would be a reasonable assumption. We don't have it, but
we should be like, or closer and closer to a clear winner, though it will take longer
and longer to figure out how to push back. And however, remember,
this is still a very artificial problem that was created to make
the quantum computer look good, right? This
is not a practical application. Then what you want to do
is to get to practical applications. And some of those artificial problems,
they do have the beginnings of scratching the surface of a
practical problem. Some of them do, some of them don't. So later, what
you really want to achieve is Can you do something a little
bit useful for somebody, even if it's a very narrowly targeted kind
of use case in chemistry, let's say. Like that'll be the next
level. And then of course the final level is, it's
when your cell phone calls up on a computer, right? That's really, it's like, yeah, it's like
a very complicated logistics thing and it just like, you don't even know it, you
type it in your cell phone, it calls up on a computer, you get a result out, right?
And that's really when it becomes useful for everybody. But there's
a lot, of course, there's a lot of things to, to achieve, at
the very end, the quantum computer has to be very cheap
for you to justify using it and very robust. The
previous step before, when it starts to be a little bit unuseful, you
can picture some big international companies,
let's say pharma or logistics, that have a lot of money to invest in these problems,
that they find usefulness for that. But we are not at the stage
where quantum computers, we use them because they're useful or they're
Anyway, we're not there yet. Yeah, not there yet. I mean, one thing that
I realized recently, or like, and I learned recently is that there's a
difference between qubits and logical qubits. Can you
talk about that? Like, like, so when there's this, whatever,
what was the most recent one, like over 1000 qubits, like
the IBM one, the one that was just released, but that that system
might not have that many, like, quote, unquote, logical qubits, even though it
Yes, yes. So remember when I was discussing that you sometimes use
many, you have a human pyramid
and you have a lot of people supporting one cheerleader at the top, right? So
it's that difference. It's like, oh, yeah, we have a cheerleader that's just
Based on that and based on what we were talking about earlier, which is that there's this no copying principle
that's like super fundamental. Like, how do we get those gains
by things other than copying? It sort of seems to
me that those logically are fighting each other in my brain. One is saying,
oh, there is actually a way that you can have a qubit that is supported by other qubits.
And then the other one says, well, you can never copy information. So in
theory, each qubit is totally independent from each other. How do those two things interact?
Yeah, so it's really about the structure of how you connect those qubits
together, such that together they're more robust than
the sum of the parts. So if you think about the
human pyramid, like sometimes you hold the shoulders of
the other person, the other to you, and you're really robust to people.
Well, if you hold a single leg, it's much harder. You have to be way stronger. So
it's really that kind of structure that you distribute the
load in such a way that together you're more robust. There's some assumptions
to get there, though. You have to be strong enough, or in
a cubit, you have to be robust enough, or quality enough, that you can help
that. Because if you're too weak, then the people above you are going to follow you. And
you cannot keep going, right? So there's some assumptions. The cubits have
to be good enough and the gates have to be good enough that you can achieve this
scalability, which is called quantum error correction. Which is, by the way,
this is the one that gets more press. It's just one
of many things you have to do to manage the errors. It's just the lowest level. There's
I would love to spend so I definitely want to get to that because I know that's what your company is
doing. So we'll get there. I think that I'm curious to hear a
little bit more about quantum error correction, though, and like physically what's
happening or like how the supports like what does the support actually look like
for like the the pyramid? Like what what are the ways that quantum
error correction actually corrects or actually helps out other
Yeah, so for quantum error correction, you need all those three axes
that I mentioned. You need enough qubits. They
have to be connected just the right way well enough and
densely enough. And then you have a certain threshold of quality for the
gates. And if you do that, you can come up with structures
of how they support each other that together, they represent one
big qubit that is really, really robust. And
the idea is if you have all those three thresholds high
enough, Once you do that, you can do two, you can do many, and
you can keep scaling this up. So it's really a transistor moment
at that level. Transistor, of course, it's above
that, just exponentially increases, amplifies stuff. So
that will be that level that we will reach, that you find the right framework
such that you can keep building them and keep adding, and they
But what are those structures or what is
the support that they're giving each other? I still don't really have an intuition for what
It's really the quantum gates that we were discussing before. It really
looks like that. You connect the qubits in a specific way with specific quantum
gates and use specific measurements at certain times and so on to
really add them all up, many like one qubit. So
it's really that you input many qubits and the output is like one qubit that
happens to be better than all of them. So it's really a
Okay. Got it. Okay. That makes more sense. So there
are certain, like basically circuits of quantum, of qubits that
together can approximate a qubit, but the circuit is more like,
it doesn't have longer coherence times or something, or it just has, that's
That's the main benefit. It's basically, it
spreads out the coherence among many qubits in such a way that it
affects less, basically. Which is really like the human pyramid, that
Okay, so let's talk about the other ways that people correct for errors
in quantum. So there's quantum error correction, which is like the kind of most
That's the holy grail. Because it needs a
lot of improvement in hardware, for example, and we
don't have a good platform. We're hopeful that this engineering problem will be solved,
but it's hard. It's still very hard to make improvements. There are there being improvements, it's
continuous improvement, but this is why when people are pessimistic about
quantum it's because Oh, well, we haven't achieved that yet
at the hardware level. And I'm not pessimistic, but that's the
absolute truth. We haven't achieved that. We can't have that
scalability yet. But there's other things we are doing now on top
of that to improve it. So another one is what's called error mitigation. There's
a lot of companies working on that. And error mitigation is really like
control techniques, like from electronics or mechanics, just
applied to quantum problems. So it's
really like when you put a
broomstick on your hand and you're trying to balance it upside down. It's
really like you move it dynamically. It's really the equivalent for that for
qubits to prevent the errors from falling apart. You
have a qubit, you don't want it to fall off, you balance it like a broomstick in your hand.
So that's the kind of thing people do with error mitigation.
This is done not necessarily with gates, although some things can be
done with gates. It's mostly done at a lower level that's called the pulse level.
So, that means that for error mitigation, you need
access to the hardware at a very low level, essentially. Basically,
you have to beg for permission for them to let you
put a box next to their quantum computer. The
pulse level, it's the gates. Remember the gates we were discussing was
like logic, right? Like ANTs and ORs and such. But ANTs
and ORs are of course made of transistors
or vacuum tubes back in the day with electricity and
so on. And they have limitations on how many you can plug in together. And you
have voltages running through them and the shape of the pulse matters.
And this ringing is really the level of the ringing of the pulse. So it's really
Change the frequency with which electricity is flowing through these things?
Yes. So really, what you really do is instead of
a gate, you really want to control that. It depends, of course, on
the application, but you really want to control it at the sub gate level,
like below, lower than the level to do this
Yeah, that seems like obviously hard to do if you're not one of the 100 people that
The techniques are very mature, but there are limitations to them. Because
sometimes when you're doing something, it affects all the qubits. For
example, there's assumptions we make to simplify things that the qubits
are not correlated. And that's not true. They are correlated. The
noise from one qubit does pass to the other. What I do to one qubit does
affect a little bit the one away from it. Of course, it's
a first order. It doesn't matter. But when you're doing pickup,
it usually matters a lot. So you do have this crosstalk between them and
Interesting. And so your thing is different than both of those. You're not
working on error correction or error mitigation. You're working on like a secret third
It's also secret, it's another problem that we call
algorithmic qubits. And these are the qubits that you can, these are the errors that
you can only, so it's algorithmic errors. These are
errors that you can only see when you actually run the whole circuit. And
the premise is the following, is that for all the things I told you before,
with error mitigation and error correction, there's assumptions that we make
so we can do the math. For example, we assume the qubit errors
are of a certain kind that are very simple
in some way. They're Markovian, for example. That's one of them. We assume they're
not correlated to each other. That what I do to my qubit does not affect the other one too
much, that it matters, and so on. And this is
true to some extent. But when you do the calculations, you
realize it's not quite true. Like what
you do now does affect the future circuit you run a little bit. And
these are called algorithmic qubits. manage them
on very, very, just doing this very fast-paced, short-term things. You need like
much longer term applications. So, the
way I like to think about it, it's like
the waves on a surfer, right? That
it's not just the average, right? Like people assume like the average is for quantum
error correction, so it's like the tidal, the tidal is high, the tidal is low. Yeah, that's pretty good,
but you don't surf the tide, you surf the waves. And you have
to feel the wave. And you have to get the right timing for
the wave. And other surfers affect your ride. Like
you see, there's fights between surfers all the time, because they do affect each other's ride, right?
If you're tired from before, that affects your ride. All these things do affect how
well you are. And you have to feel it has to be a very dynamical back and forth.
And I think that the way we do quantum computing now, in some
way, all quantum developers that know enough physics They do
sense this, like they do it a bespoke way. For example, when
you submit a circuit to quantum hardware, and the
quantum hardware is calibrated to, and they tell you what are the error
rate parameters. These are very approximated, the errors are not,
they don't study the errors as complex as they really are, they give you a
rough estimate of the errors. And so then they calibrate
the computer and the ledger starts submitting jobs. So there's people,
there's a whole lore. It's a little bit like wishful thinking
or like boxers that don't like to fight on their blue moon or
stuff like that. Crazy stuff like that. So there's some people that say like, oh, I
better submit early in the calibration window because the cubits are the
best. They're fresh out of the oven, you know what I'm saying? But
then there's some people saying, no, no, maybe I want to wait for the middle, because then
they have stabilized, and when I get statistics, they're more consistent with
each other. And this is really algorithmic errors, like screaming, look at
me, look at me, why are you ignoring me? Of course, what you really
want to do is, no matter when you submit the job, if
you want to do an offset, you want to understand the errors well enough, then
you can do You can do a data analysis
at the end to put it together. So you have to analyze the errors at the beginning, do
the run, and then do specific mathematics to aggregate
all the errors in the clever way that you don't wash out stuff.
And that's really what we do. That's really what we're focusing on.
We have made some scientific discoveries for
specifically understanding the most complicated kind
of errors, and we can add them to existing code off
the shelf. to help it improve its performance on
How much of this is like algorithmic or like mathematical laws
that you've sort of discovered versus just like raw
statistics of like, we've seen a million of these trials and we know that
they tend to be like this. Like, do you, do you understand those errors
down to the like fundamental physics reasons they're happening?
Yeah, so there's a whole continuum between those two extremes. So
if you try to understand it fully mathematically, then the
cost of understanding is so high that you don't get much
performance from the help it gives you. And
of course, on the other end, if you're too naive, then you don't get good
performance at all, right? So what we're trying to do is really get the sweet spot of
this. And for this, we do use basic
statistics techniques, such that from
a little bit of data, we can predict what's going to be the next thing, and we can suggest
ways to improve the next few rounds of the circuit. And
then the end user gets the results out. Let's
say a finance guy. A finance guy looks what a good finance portfolio looks
like. I don't. or a pharma guy that knows how to go product looks
like. And then they want, always what they do is they run something and
it's never good enough. And they want to try slightly different parameters. So
we allow them to improve those knobs to then have it
run again with that. And of course, as we're doing that, what we're really
doing is really gathering a lot of data about how the quantum computer really
works. And that allows us to take some shortcuts such
that we spend less and less on characterization. So ultimately,
the more users we get, on the
same hardware or with the same program either it can be the both are better but
it can be either one then the more performance we can provide and we
really think that this is this is always going to be an element that's very different
of quantum development that does not exist in classical developers
classical developers like just write a code and like it's done
like you just like compile it and it's done it's like there's not this dynamical element
Because it's really like a train, ultimately. It's on rails. And
piloting is very, very dynamic. You always have to work around the storm. So
our thesis is really that quantum development fundamentally is
different. We have to think about it different. The workflows have to be different. And
Fascinating. So is this a tool that runs only
after each run, basically? So like you do you do a one pass
through the all the algorithms run, you get your answer that you're like, the answer wasn't quite
right. Let's do something different the next time. Like that's the that's
the place where it inserts is not like a process that happens during the
calculation is something that happens to help you set better parameters basically
Yeah, so when you submit a quantum circuit, remember you have to run it
many times to do statistics on it, right? So it does it
in between those, and it does recompile the circuit live, essentially,
using some specific techniques for that, such that it's like, oh,
I learned a little about this error, let's just switch around some qubits because it's
a better way to work around this error. And so you do
get some statistics, then after you have compiled enough, then you can come up
with other clever solutions you can do, and you can keep improving and
improving. With that said, there are some patterns to
most quantum algorithms that we can reuse over and over. I
told you there's always the layer of tackling things, the layer of measuring things, they
always look the same. So there's those solutions that just, like once
you know for one, you can be, they're very cheap to reproduce for others,
some of the parts. Not all, but some of the parts are. Yes,
so there's different modalities of quantum computers. We're
focusing on all the ones that are gate model based, the
gate model. There's some others, for example, we didn't go into them, but there's
annealers, for example, there's photonics. They use slightly different
way to specify the problems. And the reason why
I'm focusing only on gate model, which are the atoms and
the superconductors that I mentioned, is not because one technique doesn't work for
the other, but because there is a standard that
we developed as a community for what is the proper format
to write quantum circuits for them, and we call it QASM. So
that's the standard that we use. And that means that if
they support the standard, then the techniques work for all of them. So
that's an advantage that this algorithmic approach
has, as opposed to error
mitigation, where you actually need to beg the algorithm to
let you into the room with your box connected. This is very, very
different. And I don't see them as competitors. I see them as complementary, ultimately. But
you don't want all of them. To have a good airplane that
flies very well, you do want to have very good
aerodynamics that works really well in the wind tunnel, but you also want the
pilot to have a radar and good weather models too, right? You want both, right?
So we see ourselves as kind of the radar, the weather model, so that
Is there an interaction, I guess, between you and the quantum error correction folks?
Like, are there things that you're learning that can help inform better error
correction? And like, like both of you working together? Or
is it a trade off? Like, okay, once we have quantum error correction, this
algorithmic stuff is like less useful or vice versa. Once we have the
algorithmic stuff, we don't even really need quantum error correction anymore. Do you see it more of
No, it's really complementary. You want all those layers to
be done very, very well for the ultimate flight. You want them
all. You want them all. And I do think that as error
correction matures, for example, the computational power
that there will still be these algorithmic errors. They're still
going to be there. There's going to be less of them. But
then the computers are going to be so big that it's going to be very difficult for them to subtract them by hand.
So you still want automated tools to do that for you. So we do think
that that's always going to be a crucial part that's fundamentally different
from how we program classical computers. There's no analog to this issue in classical
computers. And so ultimately you
So we mentioned really quickly at the beginning, the difference between like before
we even started recording about probabilistic computers versus like quantum versus
classical. I would love for your take on just like what those are
Yeah, so I was telling you, so classical computers are like, if you take
the globe or the Earth, it's North Pole and
South Pole. Parabolistic computing is if you could use the information in the
whole axis between the North and the South Pole. So that whole line. So
it gives you more. It does give you more. But it's not quantum. It's
not quantum. It's fundamentally, it gives you like,
there's like this, this is where I trust my complexity
theory strength. You don't get a
significant advantage on an hour. In practice, that
doesn't mean much. It could be that you get in practice a good advantage, ultimately. But
in principle, from the complexity point of view, it's kind of the same category. It's
a little bit like trains versus
motorcycles. Like, yeah, it's like, yeah, I mean,
both are kind of neat and they're different. Like you do one, like there's, you
can go to the motorcycle places you're going to go with the train. The motorcycle is
not an airplane. It's like really, really different. So
I see them like that. It's like, it's like, it's nice. I, I think
that ultimately we, we need many kinds of computing for
so many different problems and there's different criteria we should use,
which is computational power is one of them. But for some problems, you
don't need all the computational power, but there might be energy advantages for some
of them. And so I do think that we're going to be, computation
in the future, because it's going to be so important for humanity, that we're going to really think on more
dimensions of what does it mean for us. Just like when,
I mean, I live in Europe, so when I'm going to go somewhere, I sometimes take the
bus, and then take the train, and then take the airplane, right? Like, that's how life
Yeah, totally. I mean, I guess to sort of like, take a
take a step back and I think about this higher level, I mean, you could be spending
your time on anything, like you could be working on the
pharma itself, or you could be working on classical computing, or you could be
working on this probabilistic stuff. Like what what keeps you so excited about quantum? And
I think quantum computing is the biggest revolution in computing since computing. It's
really that much. I got interested in
it when I was a teenager in Puerto Rico. I just loved asking
questions about the limit of computability, what does it mean for the mind, what
does it mean for reality and such. And very quickly, I was just obsessed
with, oh, quantum computing, that sounds like the thing to do. I've
always been very interested in history, the history of computing. And I'm like, oh
my god, that's going to be a big thing. So I
used to be a computer engineer back there. And I decided, like,
after I finish my degree, I'm going to study physics. I'm going to do grad school in physics because I
want to be part of this field. And everybody, all
my professors in Puerto Rico were telling me, you're crazy. This is insane.
Those computers don't exist. I'm like, I know, I know. That's why I want to work on
them, because they don't exist. I want to make it happen. And so my background
really has been focusing on those areas, like really the hardest problems for that. And
I do think like there are some problems for humanity, like chemistry
and finding new materials that are going to be transformative for us
or for the future. And this is a way that's going to
be a big, big revolution for all humanity. So I want to
Totally. And how soon is it coming? I mean, I feel like quantum
computing is one of those things that's absolutely gone through the waves. You know, it's been the coolest
thing in the whole world. And then people are even talking about quantum winter
or something like, oh, it's out of out of fashion now. So I
mean, for a true believer that believed in it even before it was cool, I'm
sure. Yeah, it's easier to weather those storms. But yeah,
Yeah, so of course there's the hype curves,
right? And I hate those. I hate those. Because if you look at the hype curve,
it's a huge curve. It goes up,
up, and then down. And there's the valley. And then it's continuously
going up. But if you erase the hype, you will see that there was always
a continuously going up heating underneath. It's just the hype is bad.
It doesn't mean the problems don't happen. The hype is what's bad. So when this
happens, I just ignore it. I know what we're doing. These are the roadmaps. This is what we have to do. It
doesn't matter. And so I, in some way, like, Of
course, this has implications for people. It's
easy to follow the buzzword, right? It's very easy. But
I think you have to be before the buzzword is
happening. So I am very committed to this field. I really love
what I'm doing. And I do think that this is absolutely the future. So
what I would tell people is, Don't get your news
just from press articles on the newspapers because they're
like, they're so bad. They're really bad. Even when there's positive news,
they made me cringe about how they write about them. Like, I'm celebrating the
achievements of my friends, but when I see a newspaper, I'm so ashamed of
how this is portrayed because it gives the wrong impression about
how this is a cumulative, continuous development that has to happen.
It makes it seem like one guy come up with something and
Thank you so much for doing this. This was awesome. I really appreciated you taking the time to chat
with us. Any final words for people or places you would send them
Yeah, so this was a lot of fun, Cristian. So yeah, so of course,
you can reach out to me at commutatorstudios.com, of course. And you can ask me
more about what we're doing to shape quantum reality. I