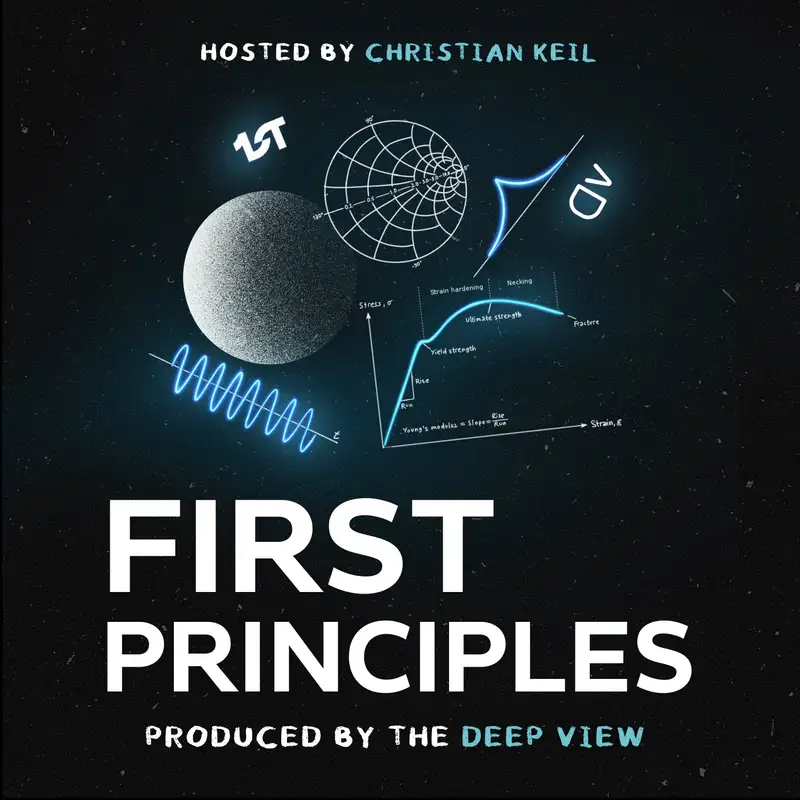
#2: Casey Handmer - Pulling Methane From Thin Air (Terraform Industries)
Hey everyone, I'm Christian and this is First Principles. Today we're talking with
Casey Handmer about the physics and economics of Terraform Industries,
a company that is pulling hydrocarbons from the sky. Casey's
first hydrocarbon is methane, which basically has two ingredients, carbon
and hydrogen. He can get the carbon directly out of the atmosphere from CO2,
and he can get the hydrogen directly out of water. So from
the raw ingredients of sunlight, air, and water, Casey
is creating natural gas. It might sound too unbelievable to
be true, but the chemistry is actually extremely straightforward. And I think that
you'll see in this episode that Casey is not some sort of crazy alchemist,
but rather just a really creative engineer. So let's hop into
it. This is Casey Hanmer with Terraform Industries here on
First Principles. Thank you for joining. And you want to just tell us
Yeah, thanks Christian. It's great to be here. I'm building Terraform
Industries. We're producing synthetic natural
Hell yeah. That is a very succinct, perfect intro.
So how did you learn how to do that? Like, I feel like, you know, engineers
tend to identify, or at least they make you in school, you
know, you're a mechanical engineer or electrical engineer or a computer scientist or
something, but your background is pretty interesting. Like you've been everything from a
a levitation engineer to working at NASA to now doing
what you're doing. So how do you identify as an engineer and how do you
Well, yeah, I think engineers are just people who make things. And about two years ago,
I came to the horrifying realization that synthetic fuels are something that we needed to
do urgently and that no one else is really doing it, at least not
the way that I thought was the correct approach. And so I set off to find
out what my mistakes were. And two years in, I think I've learned quite
Was the, was the original thing that you did, the paper, like, did you start by
thinking through it and sort of like written paper form of like, how
do I do all these steps? And will it actually like, does the engineering study close
So it kind of, I knew, I knew even 10 years ago that the key was something to do with energy, but
as these things go, you know, often by the time you get to the destination, it
seems much more obvious than it did along the way. And I guess the major intermediate step
was that I wrote a book about synthetic hydrocarbon. I wrote a chapter. about
synthetic hydrocarbon supply chain in a book about industrializing Mars, which
is, you know, one of my all-time best sellers. And that's a slight joke,
by the way. And then, you know, I kind of came to realize that, you know, kind of asked
backwards, that energy is the thing that underpins our civilization. We think
of ourselves as being in the Iron Age or something, maybe the Silicon Age, but really we're kind of in
the hydrocarbon age. Everything that we see, do, eat, you know, live
with, et cetera, depends on fossil fuels almost exclusively, well
in the majority. of our energy comes from fossil fuels. And so we need to
solve this problem. And then at the same time became aware that we were getting really,
really good at doing solar power and that solar power was getting cheap
enough that you could actually, instead of burning fuel to make electricity, you
could use electricity to make fuel and still not lose money.
And so that was kind of the germ of the idea. And obviously the precise
components or the precise details of the technical implementation still
had to be worked out. But I kind of started from that perspective and worked my way through
and spent probably about a year doing experiments in my garage and reading
and analyzing and trying to get a handle on some of the heuristics necessary
to design the process that would work in the real world. It's kind of like you
start with this infinite sea of possibilities and you have to build a couple of
bridges into the unknown so you can think sensibly about it and understand where you
You became aware that this was a very urgent problem, that something needed to happen immediately. What
Well, first of all, there's no other way of solving the climate problem, right? Maybe 10 or 15 years
ago, I was kind of disconsolate and disappointed, as many are, that our political system
had failed to solve global warming through Kyoto Protocols or
the Paris Accords or COP26 or whatever. And as
an 18-year-old, I thought, well, yeah, we should just stop using oil. And then gradually, over
time, I came to realize that just stopping using oil wasn't an option because you
know, the lives of most of the world's population depend on oil, and
that's not hyperbole. Like, if we tomorrow found that we could not use oil anymore,
within a year, a good fraction of us would be dead, and within 20 years,
almost all, as it stands today. And yet, we are in a situation where in 20 years'
time, we'd better damn well not be using any more oil, or we're going to bake our grandkids. So,
kind of in this impossible situation. But it's not just a political problem.
It doesn't matter if you live in a representative democracy like the United States or an autocracy
like China. There's no way for a political system to impose abrupt
cessation or even a meaningful reduction in the use of hydrocarbons because they
get voted out. They get voted out even in places that don't have elections. They
would get voted out as autocrats discover from time to time. It
can still happen to them. And so instead, the way out is, well,
you need to make more energy, you need to make it cleaner, and you need to make it cheaper. This
is not exactly revolutionary thinking. And certainly
we've seen enormous strides in the deployment of solar, wind, and batteries
over the last two years, really kind of hitting the mainstream. And that's basically just
that story. It's not driven by subsidies. It's the fact that this
is just cheaper and money has this way of finding the cheapest option
or the most productive option. But there's still kind of this missing piece, which is, sure, we can electrify
the obvious stuff. We can electrify our buildings gradually. We can electrify our cars.
I'm sitting in an electric car right now. The vast majority of the world's fossil
fuels are used on on extremely necessary industries
that have very low revenue per ton of CO2 produced. And you
can't just, you know, cough up a thousand bucks a ton to capture that CO2 and stuff it
underground when you're done. There's not enough money available to do that. There's not enough wealth in the entire
world. So you have to find some way of actually making
a substitute drop in hydrocarbon fuel that's cheaper than
drilling a hole in the ground. Unfortunately, this is not all that hard. It's certainly hard, but it's not
impossible because, you know, drilling holes in the ground is actually quite difficult. So
Absolutely. So what, what is it, what's so magical about hydrocarbons that,
that made us use them in the first place? Like maybe what, what is a hydrocarbon and
Yeah, well, you'll show us about first principles. So like from first principles, uh,
humanity drives energy by kind of allowing energy. So
it drives useful energy by allowing entropy flows to, to be tapped. Uh,
and it just so happens that the humanity lives on a world earth. where there's a
significant chemical imbalance between certain kinds of minerals that occur
in the ground and the atmosphere. Well, I mean, essentially entropy being stored
over hundreds of millions of years by plants. But in
more plain language, there's a shitload of oil and gas and coal underground. and
there's a lot of oxygen in the air. And if you bring the two together and warm them up a bit, they burn and
it creates heat. And with heat, you can do almost
anything. Our bodies create heat, but a couple of hundred watts to
keep us warm. And as we run around, but there's just not that much you
can usefully do with that much energy. And we
saw that in the limitations of pre-industrial societies and their persistent and
I think axiomatic inability to raise all but the tiniest fraction
of humans out of grinding poverty. And yet, once we started burning coal
and oil and gas, actually, it became pretty straightforward to
buy almost everyone a pretty good quality of life. And by pretty good quality of
life, I don't mean we're all flying private jets around, but I mean that most
of us have not experienced our children starving to death. That's certainly something that people
take for granted today, but was not the case 200 years
Absolutely. I mean, I definitely don't think people know that. Like, it's very
much the bad guy now, right? Like, the hydrocarbons are causing global warming.
It's true. Well, which is true. But from a first principles perspective,
it doesn't have to continue to be true. And that's kind of like the bet of a company
like yours is that there are ways to make these things that don't necessarily lead
to bad things. Like, hydrocarbons themselves are not evil. It's just that,
you know, when released in the ways that we have released them, they do
Yeah, of course. Well, to be clear, there will still be negative environmental
externalities associated with burning or making hydrocarbons synthetically,
even through our process, because it's positive some,
but it doesn't mean there's no negatives and no downsides. And
it's also the case that if CO2 in the atmosphere did not cause
global warming over a very long time scale. Actually, the extraction and burning of hydrocarbons,
provided that you are, you know, moderately sensible about environmental impacts, you
know, not poisoning groundwater too much, etc., etc., would actually be
seen as a universal moral good, just as putting cheap
food on the table of hundreds of millions of Americans is seen as
a universal moral good, or, you know, making the internet work better is
seen as a moral good. It just happens to be the case that Very,
very, very gradually over time, we've built up the CO2 in the atmosphere to
Can you tell us a little bit, just like what are the steps of the process that lead from
Yeah, of course. So, I mean, there's many ways of skinning this cat. Many
ways to start with essentially generic inputs and end up
with hydrocarbons or useful energy products at the end. In our case, step
one, from the first moment, we're trying to find a method that is the cheapest, simplest,
easiest, most scalable approach possible. And so that says,
well, you want to minimize as much as
possible any marginal requirements on the system. So you don't want to have to
be next to a cement plant or next to a coal plant or something. So
really what that means is we need to be able to get our water and
our CO2, which are our sources of hydrogen and carbon, out
of the air. And it just so happens that everywhere on earth there's enough
water and enough CO2, almost the same CO2 everywhere and enough water
everywhere, to do this process. And then the other missing ingredient
is energy, and you need just a crap ton of energy, just like
insane volumes of energy. Of course, we don't think about it this way, but the
energy content of hydrocarbons is incredibly high. Gasoline
is a similar energy content to pure lard or something
in terms of what we do, but also gasoline
is about a hundred times cheaper than food on a
per unit energy basis. So we think, well, we put 80 bucks of
fuel in the car this week and we put 80 bucks of food into our family today,
you know, like, gee, my family's hungry, but actually the car is consuming much, much
more energy than the family. It's just cheaper. All
right, so to get to the point, we have three
core subsystems as part of our process. There's
a system that captures CO2 from the air, so its job is to take CO2, which
is about 420 parts per million, and then concentrate that up
to about 95-98% purity, thereabouts. It
doesn't have to be perfect purity, but it does have to be like you know, at least Mars
atmosphere level of CO2. And then the
second part takes water and produces
hydrogen from the water using what's called electrolysis. So
it turns out if you put enough electrical current into water you can rip the
hydrogen off the oxygen pretty effectively. And
actually that's how hydrogen was made industrially until the 1950s
when steam methane reforming took over. And then the third
step is actually quite similar to steam methane reforming only it goes in the other direction. It's
a chemical reactor, it's not a nuclear reactor, it's a chemical reactor that takes in CO2 and
hydrogen. and produces methane and also some water as
a byproduct. And the really neat thing about that is that, first of all, this is synthetic
chemistry. This is old technology. It was discovered in 1896. It uses
a fairly standard catalyst. It's relatively well understood. Similar
chemical plants have been around for 100 years. And then the reaction products
are methane and water. So unlike natural gas, which comes out of the ground and
is often contaminated with CO2 and
hydrogen and helium, actually it's a major source of helium, which is not such
a big deal, but also a crap load of sulfur chemicals
and also light petroleum fluids and other things. We
don't have to worry about any of that. So we just condense out the water and
then maybe scrub out a bit of leftover hydrogen or something and we're good to go, which
is super cool. It really makes
our lives easier. Yeah, and then the
product out of the reactor is natural gas, which is, from
a chemical perspective, essentially indistinguishable from what comes out of your gas
supply in your house and you can use to cook your food
or heat your house or whatever. The only difference
is that because it came out of the air, there's a little bit of carbon-14 in
it. So, you know, like your olive oil or like your food. With
a sensitive enough mass spectrometry you could tell the difference, but other than that it's chemically
Did you know that there are companies out there that only make money by selling your data?
They're called data brokers, and the data that they broker are things
like your name and email and phone number, and even the names of
your family members. They literally sell your information to scammers
and spammers. They are the bad guys. But luckily, there are
good guys, like the sponsor of today's episode, Delete.Me. Delete.me
is a service that helps you keep your personal information private, and they're
actually helping me do that right now. All I had to do was go to their website
and fill out a little questionnaire, and they've now removed my information from literally
hundreds of data broker websites. And I know that this is anecdotal and
it's just me, but I have seen a decrease in the amount of spam calls I've
gotten ever since I used their service. You should sign up for Delete.me to
help protect your personal data. And as a listener of First Principles, you'll
actually get 20% off a U.S. consumer plan. All you have
to do is go to joindelete.me.com slash FP20 and
use the code FP20 at checkout. That's joindelete.me.com code
FP20. Check it out. That's awesome. So the
other component of it, though, is that you do need energy to begin. Like, no energy is
free. You can't just conjure up this from the earth. So
Yeah, we get the energy from solar. So
essentially it's an energy product, and we want to be able to pass on
the cost, if you like. We'll pass through the cost or the value of that solar to our
customers as cleanly as possible, and that means aggressively
drive down all the other ancillary costs so that essentially the customer, when they buy
our natural gas, 80% of what they're paying for is just the
solar energy that came into the front end of the system. And
it just turns out that solar energy is the cheapest energy that humanity's ever had access
to. It's astonishingly cheap. To put this in perspective,
and again, this is something that most people don't fully realize or don't fully
appreciate. I earlier mentioned that megajoule
for megajoule or calorie for calorie, gasoline's about 100 times cheaper than
even cheap food. But it turns out that solar panels
and solar batteries and motors and whatnot are about 10,000 times more productive
energetically productive per unit area than agriculture. So when
we think of energy infrastructure, We
normally think of nuclear power plants and power lines and gas
turbines and stuff, but we don't normally think of the insane
industrialized agriculture that we do across the United States, particularly
in the Midwest, as energy infrastructure. But that's what it is, right? It's
a solar power plant in the form of corn and soy
that captures sunlight and converts it into useful chemical fuel.
And that chemical fuel, some of it goes into us, some of it goes into our animals, and some
of it goes into plastics and other industrial processes. But
that's essentially what it's doing. And over the course of a season of
growth, the plant successfully captures the
equivalent of a couple of millimeters of carbon out
of the atmosphere, which is quite impressive in the sense that it's
a living thing that grows from a seed. The seed
contains a little tiny packet of DNA that builds a self-replicating biochemical
robotic machinery, but on the other hand it does have
to spend an awful lot of the energy that it captures from the sun just staying alive and fighting
off pests and not getting too hot and not getting too cold, and actually
relatively little of it comes out in useful biomass for
us. So the long and the short of it is that if you,
let's say you Let's say that you
have a vision for a civilization where 99% of the
energy is in the form of electricity or gasoline or whatever, and 1% is
in the form of food. People
can consume 100 times more energy than they personally eat, which
is a pretty good quality of life. the
total amount of land that you need to devote to solar versus
to agriculture is not 100 to 1, it's actually 100 to 1 in the other direction. It
depends on your climate, right? It depends exactly on where you are, but roughly 10 to 1 or 100 to
1 in the other direction. So actually still, most of your land is growing
food and growing plants, and some small fraction of your land, usually it
doesn't even have to be like It can be arable land, it can be desert and
brown fields or swamp or whatever. You can throw some solar panels
out there and with very, very minimal attention and maintenance, they
will pump out more energy in a day than the plants pump out in an entire season, which
is super cool. If this was not the case, humanity
would be screwed. If this is not the case, even if it was cheap enough, humanity would
not have enough land to put out enough solar power to give us the
quality of life that the market demands. And that, you know, politicians
by hook or by crook will not be able to take away from us and probably wouldn't
It is. It seriously is. I mean, I think that, um, I
think, yeah, people definitely don't understand that. I think that, um, one of the things that
comes across, so if you, if you go to your white paper, like if you read the Terraform white paper and
you see like at the end of the paper or whatever, it says, you know, what
we want to do is effectively just convert an amazing amount of
land. Like we need to, I forget the exact number, but like how much more solar do
you want to make? Like some absurdly high number of, uh, or
like absurdly high, uh, increase in the amount of solar that the world
Well, I mean, in terms of like the amount of solar that the world is capturing, most
of it will still be in plants, right? Most of us will still be in agriculture by area.
We're basically, we're prepared to scale up to 400 terawatts.
Now 400 terawatts is enough enough
energy that we'd be able to provide U.S. levels of oil and
gas supply at U.S. current prices, or better, to every
man, woman, and child on Earth, which is probably four
times more energy than humanity as
a whole could ever possibly hope to enjoy just from fossil extraction. That
just isn't enough. Even with the fracking boom in
the United States, there's just not enough oil and gas underground to give the
relatively undeveloped billions in Africa and South and East Asia and
South America, the same level of energy consumption, same quality of
life that we enjoy here in America. But let's say we can obviously do that through
synthetics and so on. So there's about 400 million of our machines
for 400 terawatts is about 2 billion acres of land, which sounds
like a lot, but overall it's roughly equivalent
to the total amount of land that is currently under cities or under roads across
the world. So that tells you that you could probably do a good quarter
to a third of that just from rooftop solar if you really wanted to. It
probably makes more sense to do it in the outlying areas of cities and towns, but
it's much, much more labor intensive to grow plants and the economic productivity is
much, much lower than solar. Again,
people don't fully understand this, but essentially the answer's there. The
only complication of using solar power is that it's not there at nighttime, which
is one of the reasons why we're seeing such explosive growth in batteries, in
the battery industry. But it's also, if
you're able to build your
capital equipment for your if you're
an industrial process, cheaply enough, then it doesn't matter.
You can get by on 25% utilization. Like I'm sitting in my car right now,
which was probably the most expensive thing I've ever bought, and
I'd be lucky to use this 5% of the time, and yet I still regard it as a
good deal, a good addition to my life. So you
don't actually need to have 100% utilization of your capital equipment, provided that
it's cheap enough that it's not really affecting the bottom line, it's not really affecting the price that
Okay, there are all these different parts. One of those huge parts of the process is creating
all this solar. But what you want to do
with that solar is not just directly turn it into energy. You want to turn it
into energy that is then turned into other energy in the form of hydrocarbons and
then have people use it. So my real question is, why
not just use it as solar power? Why do we have to go through this intermediate step of
Yeah, it's not either or. I mean, like, obviously we're continuing to
develop, and by we I mean humanity, is continuing to develop solar
and wind and batteries, but mostly solar and batteries now, at a
fabulous rate, and that will continue to basically grow at
whatever the bottleneck is right now, it's grid distribution capacity, but in the
future, the new future I suspect will be battery availability, to
provide people's primary electricity needs. And also, I
would expect to see an ongoing transition away from hydrocarbons towards
electricity for applications where that makes economic sense, which includes mostly
cooling, computation, energy generation, obviously,
like electricity generation, and ground transportation. But
at the same time, hydrocarbons remain an enormously
important energy source and also chemical source for
major industries. Chemical industry is one of the obvious ones, obviously. Aviation,
shipping, And
then a bunch of other different things. So I think it would be wise to expect that
we will see pretty steep growth in electricity consumption
and production, and that will not translate to
steep decline in hydrocarbon consumption. I
think you could definitely say for sure that hydrocarbon consumption for
ground transportation will probably enter a decline
sometime this decade, from which it will not recover. But overall, hydrocarbon
consumption for aviation will continue to rise, and I hope it will rise steeply. Not
just because I'm talking my book and my business will be selling sustainable aviation
fuel, but also if you think of... hydrocarbon
consumption per capita, aviation is one of the most intense uses we do with
it, and so you're like, well, clearly we should just ban aviation. Or we say, actually,
as a function of its utility, a gallon of hydrocarbons
burnt in service of aviation is almost certainly more more
productive or has better outcomes in your life, provided
that your basic needs are being met, than a gallon
of aviation fuel, sorry, a gallon of fuel being used to have you
sit in traffic or something like that. And as someone who is
lucky enough to fly home to Australia, you know, semi-frequently, I
think this is a privilege that we should strive to
extend to as many people as you possibly can. I think that a
good future for humanity is a future where people can fly Not
just the richest 10 million people on Earth, but essentially anyone can fly anywhere
on Earth multiple times per year, multiple times per life, and
a good many of them at supersonic speeds, which is also extremely
fuel consumption heavy. But yeah,
we'll never get there on fossils. We'll never get there on fossil fuel. There's
just not enough of it. Aviation right now
is about 2% of global fossil fuel consumption. So
maybe we can get to 10x that, so we get to 100 million
people flying routinely. But we'll never get to 8 billion on
Yeah. Okay, let's shift gears a tiny bit and talk
a little bit more about the business side of things. Basically,
how is this possible to do? Sounds great, let's do it, go
build all your solar, but at the end of the day, if it's not producing more dollars at the end
of the machine than it took to create the machine, then we couldn't scale it up that high
at all. They can't scale at all, yeah. Capitalism will
punch you in the face. That's right. I
think the thing that's interesting is that this has just become
possible, it seems, from me. Do tell me if that's wrong, but it seems to
me that solar just got cheap enough. The IRA,
that just made it possible. The revenue was that much higher from
this sort of thing. It doesn't seem like it's been possible for very long.
The IRA will accelerate us for about eight years, which actually
makes a big difference. So an extra decade of emissions right now is about 500 gigatons
of CO2, which is about
2,000 gigatons of excess CO2 in the atmosphere right now. So another
500 on top of that is not great. So the IRA is definitely pulling
in the right direction. And actually, I should say, for
the historically curious in your audience, the
synthetic fuels have been produced at pretty large
scale for almost 100 years, mostly derived
from coal. So you can take
coal and convert it into gasoline. But essentially, as
I said, the chemistry that we're using was invented in 1896, so
it's been possible to capture CO2 and
to make hydrogen and turn it into synthetic fuel at scale since then. But
the limiting factor is the availability of cheap electricity
on the input side, and solar has only gotten cheap enough to make that worthwhile
in the last couple of years, and I think increasingly
so in the next couple of years. Two
years ago, I was like, well, solar right now is not really
cheap enough for us to compete without any subsidies worldwide. There's
a few places on the Earth where fuel is so expensive that we could make go
of it. But actually, the important thing
to realize is that solar is coming down in cost 10% or 15% per year. And
at that rate, you can miss on cost by quite
a bit this year and in five years' time. Nothing to worry about. And
then, of course, that cost decline is driven by increased demand and
a learning rate feedback process. like
large-scale hydrocarbon synthesis hits prime
time, that will increase demand by about a factor of 10. So
the cost declines of anything will accelerate at that point, which is, you
know, it's feedback, right? This is capitalism doing what capitalism does
best. Without cheap solar, you know,
basically solving the climate problem is impossible. With it,
it is inevitable. You know, like that's the key
That's awesome. So what, what is it that has caused the, the
solar or the cost of solar energy to come down so much? I mean, it's, you said,
I mean, you hit it at it, but I'd love to chat about a little bit more, which is, it's not
like we've invented new chemistry or it was like a
new way to make like photovoltaics. That's like suddenly 10 X
cheaper, like 10 X more efficient. Like we're actually, what, what, what
efficiency are you assuming? Maybe like 20% or something efficient on a solar cell?
I don't actually care that much. Um, cause I buy it by the megawatt. Um,
yeah. So, The land is not the expensive part. Even
though we're using a lot of land and even though if I want to buy my neighbor's
house, it's going to cost a million dollars. That's
just because a lot of humans around and because most humans live
in cities, we think that the world's covered in humans. It's not. It's just most
humans live in cities. And in LA, you don't
have to drive very far and you're like, not only is no one here, no one has ever been
here. pre-Clovis people
coming across 14,000 years ago, it is unlikely that a human has
ever set foot in this particular patch of desolate, miserable
desert. And that's
why there's plenty of land. This kind of
applies across the board, and we're familiar with this in the context of Moore's Law, with
transistors and things like that, but actually any manufactured product, which is
to say any product that's produced in a factory, this relationship
was discovered in the Second World War. But essentially over time, as production increases,
the price tends to come down. So if you plot the
logarithm of of the cumulative production on one axis and
the price on the other axis, then they'll tend to follow a straight line,
and the steepness of that line will vary depending on what sort of product it is. Typically,
the more complex products will decline less quickly, and
then the simpler ones will decline more quickly. But for solar, it's actually relatively
simple to make it, and the
learning rate for solar is probably around 35%, which is to say
every time that we've doubled production, the cost comes down by 30% or 35%. And
right now, we're doubling production roughly every two years. which
again is like a number that's hard to get your head around but you know in
2022 humanity deployed about 250 gigawatts of solar power worldwide
which is equivalent to one megawatt every two minutes and last year we deployed
about 450, 450 gigawatts which is almost twice as much. A
little bit of that is like you know things
getting back up to speed after COVID and so on but like if
I had to bet I would say that you know 2030 we
will have another year in which the relative increase is more than that
relative increase. You know, I just think that we're getting really good at this
now. So last year is about one every one minute, one megawatt every
one minute. So yeah, so as the production increases, as
demand increases, the
cost comes down. When the cost comes down, demand increases. Now, it
does not guarantee that that your price will therefore go
to zero over a long enough time period, right? It may be the case that the
price comes down, but not by enough to induce enough
additional demand to cause the price to continue to come down. But
it could converge. But in the case of solar, it is definitely not converging. And
despite what everyone has been saying about, oh, solar demand will definitely converge this year,
I think that it will probably not converge for probably another 15 or
20 years at this rate, which is both terrifying and very exciting because
If we miss by an order of magnitude, then we'll end up paving the entire world with solar panels, which would
be pretty cool, but also like, hmm, is
this really what we wanted to do? So it'll
be interesting to see. I think at that point, it's very hard to predict exactly
what will occur. It is highly likely, for example, by that point, that a
lot of the most power-intensive applications will be not done on the surface of
Yeah. So we hinted at it before, but you want to
say a little bit more just about like what the IRA is and what it did, like
how it, you said that it accelerated the advent
How? Well, so the Inflation Reduction Act contains
within it, you know, thousands of different incentives
that are mostly intended to
help American manufacturers reshore production after
three or four decades of neglect. And
I won't get into the kind of finer geopolitical aspects of this right here, but one
of the little tiny dusty corners of the IRA contained essentially
incentives or production tax credits for green
hydrogen, for green electricity, and for carbon
capture, essentially. And it
just so happens that the business that I started is
involved in all three of those in a way that It's already
extremely economically productive, and so the Inflation Reduction Act credits, which
are intended to improve economic productivity, really give
us a huge kick in the ass on that front. In
particular, the 45V production tax credit for green hydrogen,
which is worth up to $3 per kilogram of hydrogen. Conventional
wisdom would have it that green hydrogen costs between $5 and $6 a kilogram,
so $3 a kilogram kind of lessens the blow a bit, whereas
steam-methane-reformed hydrogen can be as cheap as $1 a kilogram. So it's
still hard to compete on that basis, but conventional
wisdom does not take into account the fact that cheap
solar has come along. Yeah. And, and so we basically said,
well, what would an electrolyzer look like if, uh,
if it was powered by cheap solar instead of if it was powered by like hydro or
nuclear power or something, it's actually quite different. Um,
and I have a blog post on this. People may be interested to call it like green hydrogen for
$1 a kilogram. Um, which really
delves into the details of how you go about doing it.
And essentially you cannot, you cannot, you cannot achieve economic
like relevancy, unless you're about $1 a kilogram or less. And
you cannot get to $1 a kilogram or less unless your power is cheaper than $20 a
megawatt hour, ideally even cheaper than that. And
there's basically no way of getting to that cost unless you're using solar without batteries. And
so if using solar without batteries, you have to have an electrolyzer that's
happy with 25% utilization, which means not only does it have to be super cheap
to build, so that the utilization fraction is okay,
amortization is okay. It also has to be happy to ramp up and ramp down without
too much silliness. And there's plenty of ways that
electrolyzers can get very silly. So that
basically takes you in a completely different direction than the last 50 years of academic research and industrial
development on electrolyzers. which me
and the team essentially correctly intuited about two years ago and
since successfully built prototypes of electrolyzers that
follow these new design principles and that will very shortly allow us
to demonstrate a green hydrogen production that is competitive with
steam methane reforming. even without subsidies. Now, you throw on top the
$3 kilogram green hydrogen subsidy and it probably roughly
triples our revenue, which makes up
for an awful lot of, let's say, execution missteps, which are almost guaranteed
Basically, it allows us to recover from accidents more quickly, or mistakes more quickly, which
Love it. So that's actually, I mean, that was one of the things that struck me when I was reading
those white papers was like, I love that you started, I think
it was that one, the one with the section that's like, here are things that are very counterintuitive,
but I will prove, they'll become obvious by the end of this paper. And
one of those was that low efficiency is actually okay. Like you don't need to
have super efficient solar cell, or like solar in general. Can
you talk about that? Like why do people normally want high
efficiency? Why is it so counterintuitive that low efficiency is
So traditionally we've converted heat into electricity by boiling water
and turning a turbine, and as a result electricity has been quite a
bit more expensive per unit energy than heat. And
electricity is also usually seen as a more useful form of energy,
more versatile form of energy. Obviously, they kind
of are turning on its head right now. And so when you look at our system,
we have many factors of production, many things that contribute cost to
our system. And one of those is labor, materials,
land, et cetera, and also electricity. And of all those different things, they're all
getting more expensive over time, as is the way of the world, except for electricity, which is
getting cheaper. And so if you say, well, how do we capture the upside of cheap electricity?
Well, you don't want to invest a whole bunch of money into trying to save electricity, right?
You want to actually invest your time and effort into
productively trading electrical efficiency to reduce your costs across the other axes. And
in addition, if you have a lower efficiency system with lower capex, that
basically means you're more exposed to the cost of electricity, which is good if the price
of electricity is coming down. And it also means that when the price
of electricity continues to reduce, we can pass those savings on to our customers because
we're sensitive to those costs. If you take the
other leg of the optimization and say, well, electricity
is incredibly versatile and useful and expensive, and we
think that the cost for electricity for the foreseeable future is nuclear, which
is probably 400 bucks a megawatt hour, then there's
no way in hell you can get anywhere near a dollar a kilogram for green hydrogen so we're just
going to have to eat that loss and we want to minimize that loss and we plan on operating this electrolyzer for
30 or 40 years 100% of the time so you know we can afford to
build an electrolyzer that that will operate you know at constant utilization
forever so we only have to turn it on once uh it will never have to like ramp
up and ramp down um and you know we can afford to
put you know a thousand bucks a kilowatt of high efficiency tweaks and
unobtainium in there because over 40 years we'll get that money back out, which
is fine if you're building a rig to sit on a laboratory
desk or something like that. But we're about how business
is trying to build something that's more economically attractive than
oil wells, drilling for oil, which means we need an ROI
measured in a couple of years at most. yeah
and so that basically means we can't we can't afford to amortize over 20 or 30 years we
have to get the money out really quickly so it has to be really cheap so again it's like you know
we sometimes talk about multiple lakes or multiple different solutions and it just turns out
that there's like two different stable attractors in this space and really the the
stable attractor of like cheap and cheerful has not really been explored uh
Yeah. So that's one third
of the solution is the electrolyzer. Is it similar
with the direct air capture? Is it similar with the reactor itself? Have
you done other things with those two that people would similarly think
is kind of counterintuitive or different than people would normally make those
Yeah, across the board. So again, if you're in this kind of energy
scarcity mindset, you will try and build a director capture system that
uses a CO2 solvent material that has a
very, very low energy of transition energy. Essentially the energy required
to switch it from CO2 absorbing to CO2 releasing state. And
this is the correct approach to take if you're in a situation where you don't have very much
power. commercially
available direct air capture machines are used in spacecraft and
on submarines and sometimes in scuba diving
equipment or medical equipment or
so on. And these systems generally do not have cost constraints, at least
not meaningful ones, but they often have really stringent mass constraints, which we
don't care about really because it's just sitting on the ground. And they often have
volume constraints. I mean, submarines have volume constraints, but we don't care about that either, because we're
literally putting this out in the middle of the most useless, worthless
land ever known. What we have is a
capex constraint. We don't really have an energy constraint either. So this is
not a meaningful one. We've built our DAC system to be very, very
energy hungry, and it's going to struggle to hit 20% of our total energy consumption, because
the electrolyzer just is so power hungry. So
it's very hard to do damage next to that electrolyzer on
the energy budget. So we basically decided, well, what if
we optimize our solvent material not for something that is incredibly
expensive but doesn't use too much energy, but something that actually uses as much energy as it needs, but
it's really, really cheap. And that pushed us in the direction of of
what's called the calcite lime cycle, which uses the same chemistry as cement. And
then that solvent material is typically less than 100 bucks a ton, maybe
as cheap as 10 bucks a ton if you're in the right place, which is
great because at global scale we need a few hundred million tons of it. And
so if I have to buy a few hundred million tons of limestone
for, I don't know, a billion dollars, I think that's affordable on
the global scale of the hydrocarbon industry, which is on the order of seven
or eight trillion dollars a year. I like to say just over the course of one hour meeting, you
and I, the oil and gas industry will have turned over a billion dollars, which
is kind of cool. Whereas if
you kind of go up market on direct
air capture solvents, you can go to zeolites or
amines or metal organic frameworks, and some metal
organic frameworks cost you $50,000 a gram. So if you're saying, okay,
well now I need I need 100 million tons of this stuff. It's going to cost you
$5 trillion or something. I'm sorry, $5,000 trillion, which
What's even the next word after trillion? Quadrillion? Quadrillion.
Yeah, yeah. Five times 10 to the 18 dollars. We
should talk about log dollars. Yeah, exactly. Which
is a lot of money, right? It's really hard to think
about a business model that can scale if you have materials
like that in it. As much as possible, we're trying to use materials
and processes where the supply chain is essentially unlimited. So
for example, 100 million tons of limestone sounds like
a lot, but humanity already consumes about 5 billion tons per year just making cement. And
obviously, we won't be consuming 100 million tons a year, we'll
be consuming a couple hundred million tons over 20 years. So
it barely even makes a dent in
Yeah, yeah. Can you say a little bit more about how the director capture
actually works? There's two steps. There's capture and then release, basically.
Yeah. There's also a secret, more complex third step. So
it's basically a cycle, right? You can think of a calcium metal atom
that's cycling through the system. It gets recycled over and over again. And
actually, it has a friend. It has an oxygen atom as its friend, which
sits with calcium oxide, which kind of goes around in a circle. And
calcium oxide is better known as lime. It's actually
the lime that's in corn chips when it's got a hint of lime. It's
a little bit of calcium oxide left over from the nixtamalization process, which releases
thiamine, which is an amino acid from the
corn, so you can digest it. But if you don't have that, then you'll actually get pellagra, which
is a vitamin deficiency. Sorry, it's an amino
acid? I don't know. Ask a biochemist. But
there's basically a little bit of calcium oxide
left over, and actually there's some in bagels as well. So it
turns out you can capture carbon with bagels. It's also the main ingredient in
cement and in whitewash, as in Huck
Finn painting the fence with whitewash. So this chemical,
calcium oxide, you can mix it with water to make what's called slaked
lime, which is calcium hydroxide. And calcium hydroxide
is a material that has an incredible affinity for carbon
dioxide. So you can basically make flakes. We
start off with calcium oxide, we mix it with water, turning to calcium hydroxide, then
we make flakes with it using a glorified pasta roller. Turns out the Italians
hundreds of years ago figured out how to deal with like sticky powders. And
so make flakes out of it, which is just
kind of got the right geometric properties for something that has to absorb CO2 over
Yeah, it's got surface area and it's also got enough weight that it won't blow away. So like a
powder will just kind of drift away in the wind. You
put these flakes into a sorption bed, you blow air through them, and
actually the density of CO2 in the calcium hydroxide, once
it's saturated, is about a
million times higher than the density of CO2 in air. So you need roughly, like volume
per volume, you need roughly a million times more air than you need rocks. So
like calcium hydroxide flakes, which is, I call them rocks, but it is rocks, it's
a mineral. So you kind of have this bed that sits there for
like two days as you blow air through it at a fabulous pace. And after two days,
it's like a thin layer, a two inch layer of calcium hydroxide flakes
is mostly saturated with CO2. And then you dump that out and
put it into a kiln, which heats it up to
almost a thousand degrees Celsius. And that process, which is called calcination,
breaks the calcium carbonate back down into
calcium oxide and the CO2 comes out as gas. And actually the calcium
hydroxide is also pretty hygroscopic, so it absorbs a bunch of water
from the air as well, so it releases that water and we capture that
as well. Or we can capture it if we need water. And
yeah, so the CHG comes out through a pipe because it's gas, calcium hydroxide goes
out through kind of a drainage port
because it's still a solid, and then it goes back into the
mixer and turns back into calcium hydroxide. So it's kind of this three-step process
of like solvent, regeneration, absorbing
That's good. I love that both of, in
two of the steps of your process, your wasteful byproduct is water. It's
very, typically you would think... Yeah.
Is that true? Because the other one, yeah, for the reactor, it's also water that comes
Water comes out of the reactor as well. Yeah, essentially all
the oxygen that's in the CO2 gets turned into water. And
then all the oxygen that's in the water, in the electrolyzer, gets turned into gaseous
oxygen and gets vented. So, like, our major waste product is actually oxygen. Like
trees. We just dig it, just like assholes, just releasing oxygen into
the air. How dare you. Yeah, and actually, in
some ways, actually it's kind
of an interesting thing like chemically speaking when you when you eat food the chemical reaction
that your body is using to convert that food into energy that your cells
use to make you walk around and think think interesting thoughts is it's
the same chemical reaction as if you just set the food on fire right you're you're
basically oxidizing the food and extracting energy obviously the
oxidization process in your body is occurring in water
rather than in air, but the amount of energy released is the same. And
it turns out that our process is actually creating a
hydrocarbon methane, essentially taking
CO2 out of the air and fixing it as a, in this
case it's still a gas, but we could turn it into a liquid if we wanted to. And it's
basically the same process by which plants take
CO2 and water, which is ultimately from the air as well, out
of the air and turn it into carbohydrate, not hydrocarbons, carbohydrate.
It's got some oxygen in there as well, to
make sugars and then make cellulose and grow. Then
of course we use sugars and stuff to grow as well. Our
process is more like the burning the sandwich. It's a bit more crude. It
involves temperatures and chemistries that plants could
not survive, which is one of the reasons why we're able to do it with such high
What I'm hearing is bagels and sandwiches are key parts of the process. Both of them can
They fuel the engineer, and then the engineer builds the machine, and then the machine, yeah. There you go.
Do you want to tell us a little bit just about, is it sabatier? Yeah,
Sabatier, he was a French high school physics teacher who
dabbled in chemistry and discovered this process, essentially
using bike parts, like bike pump parts, because back in those days, bikes were
the latest thing. and published it, and
then ultimately won the Nobel Prize for work related to this, which was using
metal ions to catalyze high-temperature, high-pressure synthetic chemistry
reactions. One of which he did not discover, but was discovered shortly
thereafter, was the Haber-Bosch reaction, which is used to fix nitrogen and
make fertilizer, which supports roughly half the world's population today.
Yay. Synthetic chemistry. Yay. Interwar German
chemical work. Don't, don't think too much about 1914 to 1945. Yeah,
nothing happened. Um, it's actually, uh, I
will say there's a fascinating book on that called the alchemy of air that, that gives a much
better historical context. And actually when I was in Germany a few months ago, I
was, I was lucky enough to go and visit the plant at Leuna near Leipzig where all
this stuff was first done. It's a, it's incredible place. A
field of dreams really for what we're trying to do here. Um, Yeah,
like how they did it. They didn't have stainless steel back then. I have no idea how they did it. Anyway, we
have a master car and even then it's still really hard. Yeah, so
the reactor is super cool. Sabatier reactor,
Paul Sabatier, he had four daughters, but I don't know if he has any living descendants.
What can I help, what can I tell you about the... How can I help you with
your Sabadier? No, I mean just... So we talked about
with the other parts of the, the other like kind of major parts of the process that there
were trade-offs that normally people wouldn't make, but you
guys decided were possible. Is it, is this just kind of like a more vanilla Sabadier
I would say our Sabadier Reactor is not particularly special. It's,
we're basically just trying to do it the cheap way, right? it
becomes quite a bit more expensive to operate it, much more than about 100 psi.
So we operate at 100 psi, even though that decreases our throughput, like
conversion efficiency. But then we just have multiple stages to
essentially take the water out and it shifts the reaction equilibrium further in
the direction of the products. We're also lucky in that our customer doesn't
require 99.9999999% purity. Because
natural gas is natural gas, right? It's full of all kinds of crap, so as long as we can
hit that fairly lax purity cutoff, we're good
to go. I
think we're just building the Gen 4 reactor right now, so we've
obviously learned quite a bit along the way. But yeah, it's going well. And
it's like the spooky part, right? The other two parts I was able to prototype in
my garage, but the reactor I was like, better hire a professional and
then build that. It's
alchemy, right? You're taking matter and you're transmuting it into other forms
of matter, right? It's just like, it's deeply weird, right?
It's like, atoms are not really very tangible, right? They're kind
of abstract. But then you build this machine and you
put in hydrogen and Actually, hydrogen means makes water in
Ancient Creek, kind of gives the story away. But you put hydrogen and CO2 in, and
CO2 is the oxidizer, which feels really fucked up. And then out
of the condenser out comes a bunch of water, and you're like, oh,
that's weird. Something magical is happening in there. And something which I will add, we
still don't understand, like the process by which the
Sabatier reaction is catalyzed. We kind of have a
It's so cool. It's amazing that we've captured it without really fully understanding it,
You mentioned Gen 4. You mentioned that you have the Gen 4 reactor.
You've been doing a lot of R&D, basically, to like build up each of these
steps, multiple versions, you know, trying to create ultimately one
final end. Electrolyze is fine on Gen 9. Gen 9, nice. Yeah.
Yeah, there you go. That's right. So what is
the path to like getting a, you know, actually tapping it?
Like, what do you call it? I don't know, hooking up to the pipeline or something? Like,
Yeah. Well, the next major milestone for us is the end-to-end demo. So
that's currently scheduled for the end of February, which is coming up pretty soon. So
if my team is listening to this, you should go back to work. But yeah,
basically the various pieces are coming together now, which is fun. This
will be the first time we've taken these three subsystems, which kind of work
independently, and then stick them together, which is definitely going to be a bit of a headache. we've
thought quite carefully about how we're going to do that. So it shouldn't be too much of
a headache. And so that's kind of a key milestone
for us. It's a bit like a static fire for a rocket or something. You're taking all the different systems, you
plug them all together. We put electricity in at one end and
natural gas comes out the other end. We take the natural gas, we hand it off to our customer,
SoCoGas, and they will write us a check for probably five
cents for that volume of gas. But you've shown that that
you've shown that this is a process that can make money, or that produces money. You
haven't necessarily shown that you make more money than you put in, which
is necessary to put yourself inside the tent of capitalism. Right now, we're still
on the outside, knocking on the door. Can we come in? From
the end-to-end demo, or after the end-to-end demo,
the value prop is much more legible. The
unknowns are essentially much more comprehensible to outsiders. It
basically becomes a question of execution and scaling up
and signing key partnerships. you're
getting to major revenue and then profitability as quickly as possible. And
we're certainly in a tearing hurry to do that. This is not kind of a slow
burn, it'll take us another 10 years to get to a usable prototype kind
of situation. This is a really
a lot of brain sweat to think about how we can suck weeks out of the schedule over the
course of the next 10 years or 20 years, because every week
Totally. How does
the handoff happen? Do you actually tap into some pipe or something? Do
How does the offloading actually happen? Basically, we
think that over the next few years, we'll be deploying alongside existing gas production
wells. in areas in California where
they're already producing gas from holes in the ground, those wells are in
decline. And so there's actually spare capacity
in the injection systems that take that gas and then purify it
and then shoot it into major transmission pipelines. And so
we will deploy in those areas. And then as those wells continue to
decline, we will ramp up production and just basically piggyback on
those injection systems. And that's probably how it will work in the United States
at scale, which is roughly 500 to 1.5 500 megawatt
to 1.5 gigawatt in size solar installations with between
500 and 1,500 terraformers converting natural gas in the field
and then gathering it together in a centralized purification
and pressurization injection hub that then
will go into pipelines. And then as far as the
pipeline operator or the consumer is concerned, no change will occur except over time
the gas will become a little bit cheaper. And
I think that's the way to go, right? The supply side is the way to solve this
problem. The demand side is like, well, we would like you to desire nice
things less. OK, good luck with that. On the supply side, you're
like, oh, we've figured out a technology which can produce more gas
more cheaply with less environmental impact. And incidentally, it's also carbon
neutral, which is kind of the nice to have on
Yeah, definitely. So natural gas is just low-hanging fruit for us,
but ultimately we're there to serve the market for synthetic hydrocarbons. And
sometimes when I'm feeling cheeky, I say there's actually a lot of research being done right now on
essentially taking the same inputs, CO2 and hydrogen, and making all kinds
of other chemicals, including formate and proteins,
fats, and starches. So it may be the case actually that in 50 years' time
it will not be the case that it's just like 1% solar and 99% agriculture, but actually a lot of
the agricultural land will be We'll
be allowed to rewild, and we'll be able to produce the
vast majority of humanity's caloric intake, including animal feed, premium
synthetic foods, etc., using a solar PV-based process, which
will be easily 100 times more productive. probably
more like 1,000 to 10,000 times more productive per unit area of land, which both
means we can increase food supply, reduce cost, reduce
climatic risk, reduce ecological impact, improve quality, and
then also vastly increase the supply of food available even
in places that we would conventionally regard as unfarmable or
inhospitable. So if your vision for humanity includes
a trillion humans on the Earth. There's no way we can get there with agriculture as we know it today, but
we could certainly get there with a solar synthetic supply
Love it. That's a perfect way to end. Any final thoughts? Any places
you would want to send people, things you want them, maybe check out that book,
Alchemy of Air, yeah, yeah. Well, I mean, if you're super smart and you want to
work with me, that's great. And if you're super smart and you don't want to
work with me, you should start a company and compete. We
look forward to the battle. But yeah, actually,
this kind of cheap solar is poised to revolutionize about
half a dozen to a dozen different industries. And I know a few startups
in these areas, but they would all appreciate the help. So look
around at the local incredibly power-intensive industries. Think carefully
about how you would run them off intermittent but incredibly cheap electricity, and
then go and do it. And the sooner we do this,
Episode Video
Creators and Guests
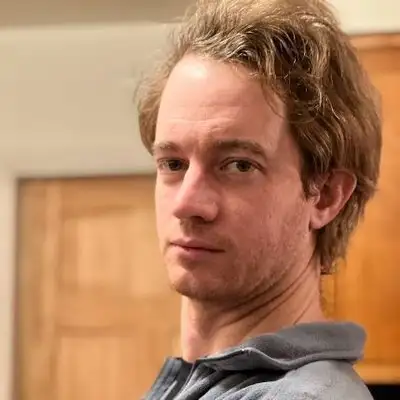